Create a free profile to get unlimited access to exclusive videos, sweepstakes, and more!
A tiny wiggle reveals a HUGE merger of black holes - the fourth ever seen
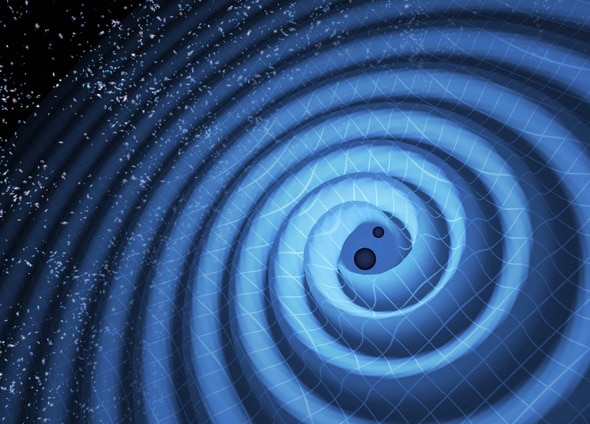
On August 14, 2017, did you feel taller?
For a brief moment, you were. By about a millionth of the diameter of a proton. You were shorter by that amount, too, a few nanoseconds later. That’s because at 10:30:43 UTC on that day, the gravitational waves from a merging pair of black holes 1.8 billion light years away passed through you, oscillating your height a few times.
I’m guessing you probably didn’t feel it. But LIGO did. And a bigger deal is that Virgo did, too.
LIGO is the Laser Interferometry Gravitational-wave Observatory. It’s actually two detectors, one in Washington State and the other in Louisiana. In 2015 LIGO detected the first ever gravitational waves in human history, one of the last predictions of Einstein’s theory of General Relativity that had yet to be shown directly.
I suggest you read the link above to get all the info, but this bit is the important one:
One of the outcomes of Einstein’s General Relativity theory is that space and time are two facets of the same thing, which we call spacetime. There are lots of analogies for it, but you can think of it as the fabric of space, a four-dimensional tapestry (three of space and one of time) in which we are all embedded. Remember, it’s not literally like this; we’re using an analogy. But it’ll help you picture it.
We think of gravity as a force, pulling us toward an object. But Einstein revisualized it, seeing it as an outcome of the warping of spacetime. A massive object distorts the shape of space, and another object moving through that warped space gets accelerated. We see that as gravity. In other words, matter tells space how to bend, and space tells matter how to move.
Another outcome of the mathematics of GR is that if a massive object is accelerated, it will cause ripples, waves, to move away from itself as it moves. These are actually ripples in the fabric of spacetime itself! Spacetime expands and contracts in complicated ways as a wave passes, a bit like how ripples will move out from a rock dropped into a pond, distorting the surface of the water.
As far as we know, the strongest and sharpest gravitational waves are created when two black holes merge, because they’re massive and accelerate hugely right before they merge. That’s what LIGO was designed to detect… and it did, in September 2016. Since that time it’s found two more mergers (plus another signal that might be one, but is marginal and so not confirmed).
What makes this fourth one special is that the LIGO observations are joined by Virgo, another observatory located in Italy. Virgo is a joint collaboration between five different countries, and originally started operations in 2007. It wasn’t quite sensitive enough to detect gravitational waves, but an upgrade (“advanced Virgo”) was completed this year.
Virgo operates along the same principle as LIGO: As a gravitational wave passes through the Earth, it stretches and shrinks the fabric of spacetime. A laser beam reflected between mirrors located several kilometers apart can measure that change in the shape of space, even though on that scale the stretching is only about 1/1000th the diameter of a proton!*
Adding Virgo to the mix is important. For one thing, having another detector means being more sensitive to the sky; weaker gravitational wave events can be “seen”. But another and potentially more important function is that it helps nail down the location of the source to far more accuracy in the sky.
LIGO has two detectors, neither of which on their own knows where the source of the waves came from. All they can do is say “I feel one!” and also tell us how strong it was (along with a few other other important characteristics). But the waves travel at the speed of light, so in general there’s a small lag between one detector feeling a wave and the other one doing so; the time it takes for the wave to move from the first to the second.
That lag can then be translated into a position on the sky, but not a very good one. That’s because the lag leads to a position solution on the sky that’s a ring, not a spot. Any location on that ring could produce that delay, so it doesn’t nail down the location on the sky. It’s like giving someone your latitude on the Earth; they know you’re somewhere on a ring around the planet instead of a specific location.
But a second gravitational wave observatory (with a third detector) breaks that symmetry. The lags between all three detectors yields a specific location (like giving someone your longitude as well). It’s not perfect, but it narrows down the area of the sky significantly. †
The location is pretty important. If the information can be sent out rapidly enough, it’s possible that optical and other telescopes can look for any sort of flash from the event, something that has not been seen yet. We don’t know if there even is an optical counterpart, but it would be nice to know where to look for one.
This latest gravitational wave event, called GW170814 (for what it is, and the date), was determined to be from two black holes merging in a distant galaxy 1.8 billion light years away. The black holes were beefy, 31 and 25 times the mass of the Sun. That’s massive.
They started out in life as extremely massive stars, probably many dozens of times the Sun’s mass each. Stars like that don’t last long, and, within a few million years of forming, they exploded as supernovae (not necessarily at the same time, but given their lifespans, not all that far apart in time, either). When each did, its core collapsed to form a black hole. As they orbited each other, they gave off very weak gravitational waves that stole energy from their motion. They slowly spiraled in, faster over time, until they got so close that, BLURP! They merged.
When that happens, a huge amount of their mass is converted into the energy of gravitational waves. How much? Well, it was the same as converting three times the mass of Sun into energy, which is a quantity that grinds my brain into dust. The energy released was equivalent to the energy the Sun would put out in about 40 trillion years. That’s 4000 times the Sun’s entire lifetime of energy output.
And this merger released that energy in a fraction of second. Black hole mergers are soul-crushingly violent events.
The good news is that it was so far away that the waves were incredibly weak by the time they got here. The fact of the matter is they’re so weak our technology is only just now capable of detecting them at all. Incidentally, astronomers looked, but found no optical counterpart for GW170814, unfortunately. It may have been too faint, or they looked too late. Or, maybe, there wasn’t one. We’ll understand this better as more cosmic black hole mergers are detected.
Finding this fourth one is another relativistic feather in the cap of astronomers, because it adds to the catalog, but even better is the news that, with Virgo online, we’re getting better at detecting them. And we’ll only improve our sensitivity with time. It’s a whole new era of astronomy, and that’s something that should make us proud, and stand a little taller.
Even if it’s only 10-21 meters taller.
* The amount something gets stretched depends on its length; a longer object gets stretched more. That’s why you stretched by a millionth the size of a proton; you are roughly 1/1000th the length of the LIGO and Virgo detectors. In reality you probably stretched less, since the waves may not have passed through you head to foot (or vice versa).
† This is very similar to the problem we had some years ago with gamma-ray bursts, huge explosions of energy that came and went so fast they were hard to locate. Then NASA launched the Swift satellite and it got a lot easier. I was on the education and public outreach team for Swift, and we wrote a classroom exercise that talks about using two versus three detectors to locate them much like LIGO and Virgo do. Scroll down to the “Angling for Gamma-ray Bursts” to see how it works.