Create a free profile to get unlimited access to exclusive videos, sweepstakes, and more!
Asteroids can reveal where they came from — and how they ended up smacking our planet in the face
It's going to come as a shock — and pressure.
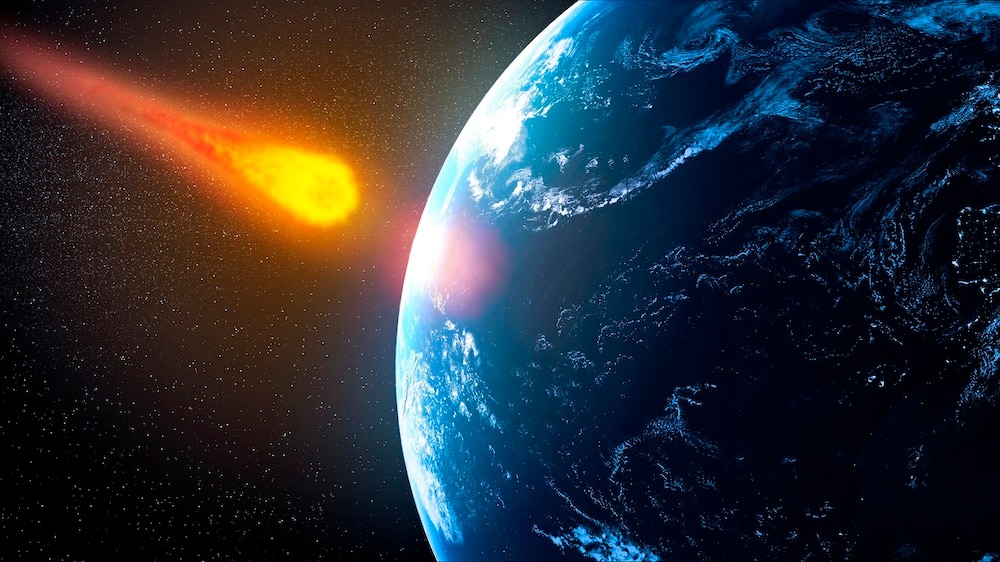
Just say “asteroid” and whoever is next to you will probably think of the thing that obliterated the dinosaurs, but rocks from outer space can be much more fascinating.
Most asteroids that plummet through the atmosphere burn up before anything the size of the Chicxulub asteroid (responsible for that infamous mass extinction 66 million years ago) can slam into the ground. Fragments of those asteroids, or meteorites, then fall to the surface and generate shockwaves. Minerals in the crust that have been changed by these shockwaves can reveal things about the rock, from its formation to its demise, going back billions of years.
How minerals deform and sometimes recrystallize can be mesmerizing up close. They might not look too different on the outside after an impact, but the inside becomes disordered. The most common mineral in Earth’s crust is plagioclase, and there have still been questions about how exactly this disorder (and possible reorder) happens within it. Researcher Arianna Gleason-Holbrook of SLAC at Stanford University, who led a study recently published in Meteoritics & Planetary Science, wanted to find out.
“Each mineral has its own unique pattern, like a fingerprint,” she told SYFY WIRE. “When this 3D lattice changes dramatically, like during amorphization, atoms pack into a new geometry. This new geometry doesn't have the same periodic structure — it becomes disordered.”
In the lab, Gleason-Holbrook and her research team were able to recreate meteoritic impacts so they could see the amorphization process in action. They could observe how the atoms were bonded using X-ray diffraction — X-rays have the right wavelength to literally shed light on that. On camera, the lattice shows up as a pattern, with clearly defined lines, pre-disorder. That pattern changed drastically when the plagioclase amorphized. As disorder happens at a certain pressure, those lines vanished into something much more diffuse. It also recrystallized when pressure was taken off.
The pressure at which plagioclase amorphizes was a mystery until now, but the researchers found out that disorder occurred at a much lower pressure than was previously assumed. Gleason-Holbrook suspects that it has always been this way. In the past, there had just not been enough technological advancements, such as X-ray diffraction and hi-res optical devices, to see the most subtle atomic changes in so little amorphous plagioclase. That the mineral can actually recrystallize after a collision was also unknown before.
“The recrystallization of plagioclase back to its original crystalline form is really fascinating — the notion of memory in crystalline structures, after completely undergoing a disordering state is not well understood.”
Amorphization should not be confused with twinning, a phenomenon that can also happen when crystals need to relieve themselves of pressure after an impact, but under extreme pressures. Twinning forms a new crystal lattice that remains attached to the original lattice. This was recently observed in a shocked zircon from the Martian meteorite also known as Black Beauty. At the pressures plagioclase was exposed to, much lower than those that would be needed for twinning, it actually chose to amorphize rather than twin.
The new understanding of amorphization and recrystallization can tell us more about a meteorite’s journey. It can show how fast an asteroid was hurtling through space, as well as the intensity of shock and pressure and how much it was heated in just a billionth of a second upon impact. How it morphed and finally cooled down can also be given away by how it succumbed to disorder and reordered itself. Minerals can be shocked artificially to match samples from actual impact sites, and their transformation which can give more insight into what happened when a particular meteorite struck.
“In the future, we hope to be able to compare more lab results with what is found in nature and look at the microstructure of debris or plumes of vapor, gas, and rocks particles,” Gleason-Holbrook said. “We could determine the pressure and temperature history of the an impact phase by phase.”
How something so potentially destructive could also be so intriguing is something to wonder at.