Create a free profile to get unlimited access to exclusive videos, sweepstakes, and more!
Astronomers find dark matter from 12 billion years ago
New method sees unprecedentedly far back in the history of the Universe.
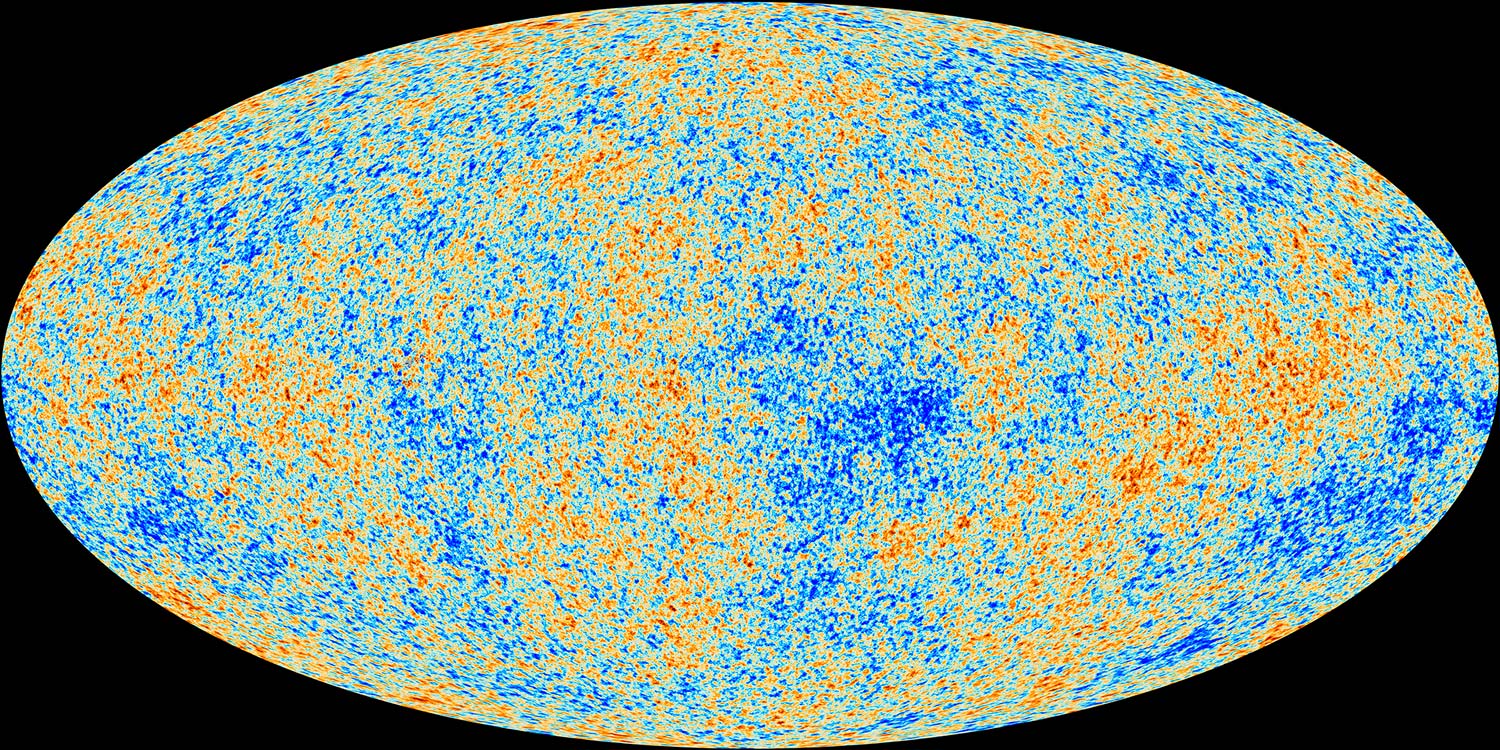
We know dark matter exists — a mysterious substance that is five times as prevalent in the Universe by mass as what we think of as “normal” matter, the stuff you and I and everything we see are made of. We see its effects through its gravity, even though we don’t know what it’s made of yet. Dark matter affects how galaxies rotate, and how they move in gigantic galaxy clusters.
We also see its effects via gravitational lensing. Mass has gravity, which warps space. If we look past some massive object like a galaxy to an even more distant galaxy beyond, the foreground galaxy’s mass warps space, distorting the image of the background galaxy. That more distant galaxy can look smeared, or bent, or even duplicated into multiple images of it. The foreground galaxy’s gravity acts like a lens, hence the name.
We can measure the light coming from the lensing galaxy and measure the mass of its normal matter that way. Then we can measure how much it distorts more distant objects and get its total mass. The difference is how much dark matter it harbors. This method has worked with great success in recent years.
But there’s an issue. At some distance from Earth individual galaxies are too faint to see, so we run out of background galaxies to use. This is inconvenient! We want to see as far away as we can, because the farther away we look, the farther back in time we see; the speed of light is finite, which means the farther away we see an object the longer it took its light to get here. So, in turn, the more distant an object, the younger we see it being.
We want to know what the distribution of dark matter was like when the Universe was very young, because we think dark matter clumped up not long after the Big Bang, and as it did it drew normal matter to those clumps, which then formed galaxies. By knowing what dark matter was like back then we can understand better how galaxies formed. Not only that, but it takes time for galaxies themselves to clump together to form galaxy clusters, the largest structures in the Universe. They’re so big that the shape and size and other characteristics of the Universe itself can be determined by examining clusters.
So how do we do that if we don’t have very distant galaxies to examine? A team of astronomers dreamed up a way: Don’t use lensed galaxies to measure dark matter. Use the background glow of the Big Bang instead.
The moment of the birth of the Universe was hot. As the cosmos expanded this fireball cooled. Eventually it got big and low-density enough that light could travel through it, and it became transparent. That happened a few hundred thousand years after the Bang, and the cosmic expansion has redshifted this glow into the microwave part of the spectrum, called the cosmic microwave background radiation. This glow was very smooth, very similar everywhere you look out into the sky. But the gravitational lensing by distant galaxies will distort that smoothness, making it clump up a bit.
So the astronomers used data from the European Space Agency’s Planck satellite, which measured that glow to high precision, and mapped it against a survey of extremely distant galaxies seen by the Subaru telescope in the Hyper Suprime-Cam Strategic Survey Program, which mapped a lot of galaxies over a staggering 300 square degrees of sky — 1,500 times the area of the full Moon! In the end the astronomers looked at the gravitational lensing around 1,473,106 galaxies (!!) whose distances could be reliably measured [link to paper]. On average the light from these galaxies took 12 billion years to reach us, so we see them as they were about 1.8 billion years after the birth of the Universe — far more distant than any gravitational lens has been used before.
They were able to determine the masses of these galaxies from how much they lensed the cosmic background, and found on average they had dark matter haloes with masses about 300 billion times the mass of the Sun. That’s actually much less massive than our own Milky Way, which has about 700 billion solar masses. But then we see these distant galaxies as they were when they were young, before they could grow to such size as our own galaxy.
They also found something very interesting. The cosmic background radiation isn’t perfectly smooth; it’s a little bit clumpy, which represents a tiny bit of lumpiness in the distribution of matter in the extremely early Universe. These clumps collapsed to form the first stars and galaxies. The standard model of dark matter predicts a certain amount of clumpiness, but the new measurements get a slightly smaller value. It’s close, but not quite the same, which hints that there may be more going on in the Universe than we think.
That’s very tentative though! The new measurements have a healthy amount of uncertainty, so it’s hard to say if the disagreement is real or not. The good news is that the big Subaru survey of the sky isn’t done yet, but when it’s finished it’ll cover three times as much sky as was used in this study. That means they should be able to hammer the uncertainties down a bit. Plus there will be better measurements of the microwave background radiation in the coming years, which will help there as well.
All of this is still pretty new; the first reliable measurement of the background radiation is only a few decades old, and using gravitational lensing method to get galaxy masses has only been around a couple of decades. This new method is another step in figuring all this out. Science is never really done; we keep refining it until we have an understanding that fits the observations and makes sense theoretically. Even then it’s not finished! There may yet be still more to figure out to make the measurements better.
That’s where we are here: Still in the middle steps of this recipe, trying to write down the basic ingredients and methods that were used to cook the Universe. The cool thing is we’re making progress. And we’ll keep getting better at understanding how the cosmos came to be.