Create a free profile to get unlimited access to exclusive videos, sweepstakes, and more!
Do your electronic gadgets have a speed limit?
It's one one-million billionth of a second.
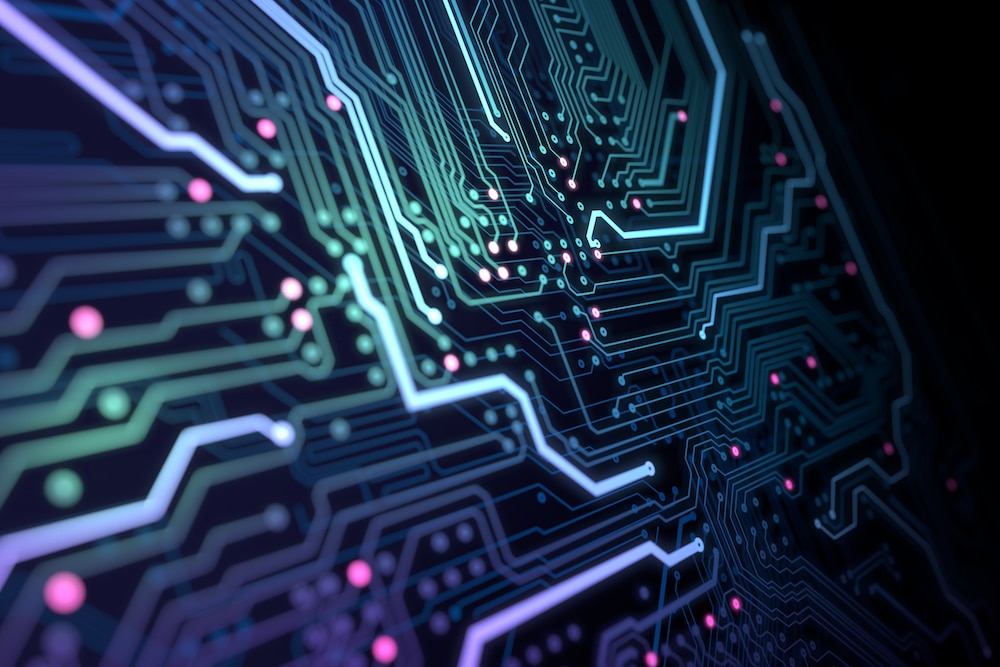
As smartphones and tablets get faster, as internet is beamed down from satellites, as 4G progresses towards 5G (which is not yet in full effect despite what manufacturers and marketers want you to believe), is there such a thing as too fast for tech?
Quantum physics says so. Researchers have now found the ultimate speed and power limit that electronics cannot go beyond — though the upside is that these extreme limits are impossible to reach with current technology, so we won’t be missing anything. Processes in semiconductors make it possible for an electric current to be generated. The shorter the signal, the less time it takes for these processes to happen, and the more instant gratification your gadgets give you. It seems like it could go on forever. Unfortunately, the potential for speed is not infinite.
What puts the brakes on something like a computer chip is actually the speed of the energy transfer involved. Zapping a semiconductor with laser pulses accelerates the electrons in its atoms. The shorter the electric current, the more rapid the transfer of energy, till electrons are pushed until that transfer can go no faster. Researchers Marcus Ossiander of Harvard University and Christoph Lemell of Vienna University of Technology, who led and coauthored a study recently published in Nature Communications, found what that elusive upper limit was.
“To induce a current, you must create asymmetry in the electron movement,” Ossiander told SYFY WIRE. “To do this, you again want to apply an electric field, which makes most electrons prefer to go along that field’s direction. But they can not always do what they want.”
So what is the ultimate speed that electricity can be transferred at? By using lasers, which are much faster and more precise than the usual transistors, the research team concluded that it could not go beyond one petahertz. That is one million gigahertz or one-million billion hertz. So you can fathom exactly how fast that is, think of one hertz as one second. Now try to imagine one-million billionth of that. Reaching it means that you have to exit the electrons in a semiconductor, and that means you have to shift them around to different places. Electrons in solids need to be moved artificially because their movements otherwise cancel each other out.
“Our signal was a short laser pulse through an insulator, driven by a second longer laser pulse,” Lemell said. “We then compared the measured current with the known electric field of the pulse. To transform the information of fast changes in the external field into the measured signal, the solid must be capable of transmitting currents at very high frequencies.”
Semiconductors are somewhere between conductors like metals, which allow electric currents to flow freely, and insulators like plastic, which block the passage of an electric currant. There are three main energy bands in an atom. The valance band is where the outer electrons hang out, and they are bound to the nucleus. When those are moved to the conduction band, which is the one ironically closest to the nucleus, they become unattached to the nucleus and therefore free electrons. They just have to pass through the forbidden zone to get there.
“One of the achievements in our current work is that we were able to create light pulses with very high photon energies, much larger than people can usually use,” said Ossiander. “We call the energy of our light vacuum ultraviolet or extreme ultraviolet.”
Pushing electrons through the forbidden energy gap usually requires some applied force. The energy in this region is the same as the difference in energy between the valence and conduction bands. There is only one problem. Short laser pulses — like the extreme UV pulse the researchers created in their lab — have a range of frequencies. As a pulse is shortened, its spectrum becomes broader. While you can make out at exactly what time the electron was hit with extra energy, you don't really know how much or what type of energy it picked up.
It is because of the conditions under which this experiment was pulled off that such a high speed could even be achieved. Ossiander, Lemell, and their team used lithium fluoride as their semiconductor, and also had hypersensitive equipment on hand to detect what the electrons were doing at any given moment. They were able to tell when exactly electrons reached a state where an insulator suddenly switched to a conductor. The fastest this happened was at one petahertz. Any more speed would have caused glitches in the signals they tried to transmit.
“It will still take a while to miniaturize the lasers and setups needed to reach such extreme time scales in millions of parallel switches required for a processor and at a size and cost that can make it into our living rooms,” the researchers said.
For just one realization of that upper limit, the space needed was about the same size (and also as expensive) as a one-bedroom apartment. Our need for speed is going to have to wait.