Create a free profile to get unlimited access to exclusive videos, sweepstakes, and more!
I’m Shocked—SHOCKED—by This NASA Photo
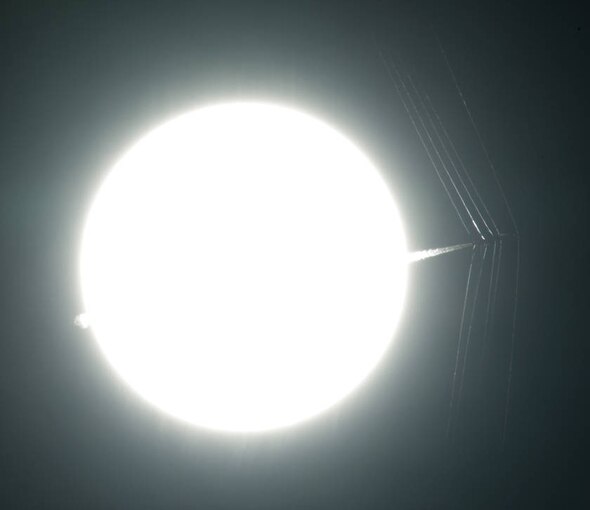
Well, that’s a weird and totally cool photo, isn’t it?
That big bright thing is the Sun. The other thing is not a telephone pole or a spider blowing in the breeze. It’s actually a T-38C jet, a plane capable of supersonic flight (it’s used to train astronauts, in fact).
So what’s with the streaks? Those are shock waves coming off the jet, captured using a special technique called schlieren (pronounced shleer-en; German for “streak”) photography. This technique allows the user to capture changes in air density, among other things.
So how does this work?
A shock wave happens when an object moves through a medium (air, water, whatever) faster than the speed of sound in that medium. When you compress air, say by throwing a ball, the air will pile up in front of the ball and then flow around it as the ball moves through it. The faster you throw it, the more the air gets compressed. The air flows around the ball in a gentle curve, called a bow wave (like the wave made off the bow of a boat).
On a submicroscopic scale, what’s happening is that the ball is hitting the molecules of air, and they get pushed forward into the molecules in front of them. They then bump into the molecules in front of them, and so on. The air in front of the ball gets denser.
Think of it like a wave of information. Each molecule bumps into the one in front of it, basically telling it, “Hey, I’m getting pushed from behind, so pardon me.” That wave can only move forward at the speed of sound due to the physics of molecular motion.
As long as the ball is moving slower than sound, the air in front of the ball has plenty of time to adapt to the compression—the molecules can communicate their motion to each other—and you get a relatively stable system with that bow wave of air.
But if the ball is moving faster than sound, the molecules don’t have time to adapt. The molecules get slammed into the one ahead of them faster than they can communicate, and the air can’t smoothly adapt. The molecules ahead don’t know it’s coming, so they’re “shocked” by this. There’s a big jump in pressure and density, resulting in a thin compressed shock wave moving away from the ball as the air is violently compressed in front and then expands away to the sides and behind it. The curve is straighter, less like a bow wave and more like streaks.
That shock wave can have a lot of energy in it, and it moves away from the object at the speed of sound. An airplane moving faster than sound makes a pretty strong shock wave, which you hear on the ground as a rattling BOOM (hence “sonic boom”). Funny, too: The same physics works for exploding stars, with their gas slamming into surrounding material at much faster velocities than the speed of sound. I did the math in grad school for a class, and it works on the scale of airplanes and supernovae.
The first A in NASA stands for Aeronautical, and one of NASA’s missions is to understand better how airplanes move through air, and make them more efficient. Schlieren photography allows researchers to easily see the shock wave patterns coming off the various bits of an airplane, and the hope is to reduce those shocks, allowing quieter and more fuel-efficient supersonic travel.
For this type of photography it helps to have a bright source behind the object traveling, and, hey, the Sun is pretty bright. The plane and camera have to be positioned just so, but this is NASA, after all. They figured that out and ran tests producing images like the one above.
I like how you can see the jet contrail behind the plane, and a little of it poking out the other side of the Sun. Had someone showed me just that left-hand part of the image my first thought would have been that it was a solar prominence! Context is everything.
I also like how, in various photos like this one, you can see the different parts of the plane making different shock waves; the nose, wings, elevator, rudder. All of these add to the problem and need to be investigated.
I’m glad NASA does research like this; you never know where it might lead, and in this case the main goal is to make transonic travel easier. Having cooled my heels on numerous airplanes flying for many, many hours at a time, this seems like a pretty good idea to me. Think of the savings just in little packets of peanuts!