Create a free profile to get unlimited access to exclusive videos, sweepstakes, and more!
The black hole on the edge of forever
Astronomers have found a supermassive black hole gobbling down matter at a tremendous rate.
"So what?" I hear you thinking. "We find those all the time!"
Yeah, but this one is a little bit different. It's 13.1 billion light-years away.
Yegads. That is a seriously long way. In fact, this quasar, called J1342+0928, is the single farthest one ever found! And there may not be too many more at that distance. This one is a record breaker, by a substantial margin.
There's a lot of coolness to be had here, but explaining it takes a few steps. Each one is small, but they add up to a very, very long way. Ready? OK, let's go.
We think every big galaxy in the Universe has a supermassive black hole in its core. They form together, and the black hole grows as it feeds on the gas and stars in the galaxy around it. As the material falls into the black hole it forms a flat disk, called an accretion disk. Stuff very close to the black hole is whirling around at near light speed, and stuff farther out is moving more slowly. That means there's a lot of friction in the disk, and that in turns means the material gets hot. Really hot: millions of degrees.
When you heat something up to those kinds of temperatures it'll glow very brightly. Accretion disks around big black holes get so hot that they are incredibly luminous, and so bright they can be seen for billions of light-years, clear across the observable Universe. Galaxies with supermassive black holes that are actively feeding and glowing this way are generically called active galaxies, or sometimes by an older but still cool term: quasars.
Got it? OK, next step.
The Universe is expanding. We've known this for nearly a century now. The details are incredibly complicated, but the basics are that at some point in the past, all of space and matter were condensed into a single point. Suddenly that point expanded. It was incredibly hot at first, but cooled as it grew. That was roughly 13.8 billion years ago, and things have cooled a lot since then.
That also means that galaxies that used to be near us a long time ago are now pretty distant. But there's a funny quirk of the Universe that lets us see some of them: the finite nature of the speed of light.
Light travels fast, but not infinitely so. When we look out across the Universe, we're also looking back in time. If you see an object a billion light-years away, you're seeing light that left it a billion years ago. So telescopes are like time machines: The farther away we look, the farther back in time we see.
But there's a catch. The light coming from so far away is traveling across space that's expanding. It loses energy as it does so, and when light loses energy its wavelength increases. A photon (particle of light) that starts off blue might shift to the red by the time it travels far enough (again, details are complicated and I'm simplifying, but this is close enough). We call this phenomenon cosmological redshift. The farther away something is, the more it's redshifted.
We're almost there. Hang on.
In the meantime, the Universe is filled with thin gas. Hydrogen, mostly. A hydrogen atom is a proton with an electron whizzing around it. We call that neutral hydrogen. But if you hit it with a high-energy photon it'll knock the electron off, like shrapnel. It takes ultraviolet light to do that.
Right after the Universe formed, it was so hot that all the hydrogen was ionized. But it cooled as it expanded, and at some point (a few hundred thousand years after it formed) it cooled enough that electrons could combine with protons, and the hydrogen became neutral.
For that time, the Universe was transparent. Neutral hydrogen is bad at absorbing visible light (the kind we see). But then something happened: Stars and black holes formed. They blasted out ultraviolet light, and that has enough energy to ionize hydrogen again — we call this period reionization. The electrons were freed, and they're good at absorbing visible light. There were so many of them back then that the Universe became opaque again. Eventually the Universe expanded enough that the density of electrons dropped, so much so that electrons were few and far between. The odds of a photon encountering one dropped so much that the Universe became transparent (again).
Here's the thing: Imagine a big black hole voraciously eating stuff around it, and that stuff glowing intensely, blasting out light at all wavelengths. That light could travel all the way across the Universe to reach us … unless there's some neutral hydrogen nearby. That'll absorb all the high-energy light and become ionized.
Now let's put all this together. The Universe is young. J1342+0928 — our record breaker — is a new black hole, and very massive. It's eating stuff left and right, and blasting out radiation. Nearby is enough neutral hydrogen to absorb everything higher-energy than ultraviolet. Everything else passes through, and travels over 13 billion light-years to reach Earth. What do we see?
Remember, over that distance the light loses energy. We see it redshifted, a lot. What started as ultraviolet light at J1342+0928 is now infrared when it reaches us! If we take the light we see from it and plot it out, the graph should show nothing at the high-energy (short-wavelength) end, because that was all absorbed, then a bump where the hydrogen lets light through, and then a bunch of light with lower energy (longer wavelengths). And that's exactly what we see.
That graph shows how bright J1342+0928 is on the vertical axis, and the horizontal one is energy, or wavelength, or color (they're all different ways of saying the same thing). Energy gets higher to the left. There's basically nothing we see in visible light (the kind we see with our eyes, on the extreme left of the graph); that's all originally high-energy light that got absorbed locally, now redshifted. As you move to the right there's a bump — that's the lowest-energy ultraviolet light neutral hydrogen will let through. Then to the right we see the normal spectrum of a hot accretion disk.
The location of that bump tells us how much the light got redshifted, and it's a lot: We say it has a redshift of 7.5, which means (due to the math and the way we measure it) the light has been redshifted by a factor of 8.54. I've done some redshift observations before using Hubble, and it was common to see galaxies with redshift factors of 1 or 2. But 8?! That's huge! This object is really, really far away. So far away that all the light is shifted to the infrared; it doesn't show any visible light at all.
In fact, that's how it was found in the first place. Astronomers used different filters to take very deep images of the sky and look for objects that are invisible in near infrared but bright farther out in the infrared — the hallmark of a very distant, bright object. That's what those two images inset at the top of the graph show; the left one is very red light, and there's nothing there. The one on the right is infrared, and there our quasar is.
Amazing.
There's more. Judging from the amount of light absorbed, it looks like the hydrogen in the Universe around J1342+0928 was roughly half neutral and half ionized. That means we're seeing it as it was when the Universe was right smack in the middle of being reionized! That's very exciting; it's really hard to get observations of the cosmos from this time, and here we have, literally, a beacon in the middle of it.
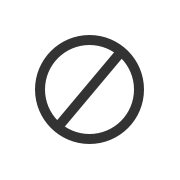
And while this is really cool and all, it does introduce a small problem: This black hole shouldn't exist.
The observations of J1342+0928 indicate it's huge, with a mass of about 800 million times the mass of the Sun. That's big, and we see lots of black holes like that within a few billion light-years of us. But black holes take time to grow. The light we see from J1342+0928 was emitted when it was very young; heck, the whole Universe was only about 700 million years old. According to the science we have right now about all this, that's not enough time for a black hole to grow to such immense size.
Oops. So there's a problem. Either it's less massive than that (unlikely, the math would have to be off by a lot), or black holes can grow a lot more quickly than we thought. I suspect it's the latter. I'm no expert, and I don't know how that might work (in my defense, neither does anyone else), but it seems the likelier scenario.
Now here's an interesting bit: This J1342+0928 is probably not typical for black holes at that era. Why do I say this? Because when you're looking in a whole new place (or time) for an object in the sky, chances are you're first going to find one of the brightest ones. When it comes to supermassive black holes, a bigger, more massive one is likely to be brighter. So just statistically speaking, this is probably one of the biggest black holes that existed at that time. It's an outlier. Astronomers estimate there may only be 10 – 100 like this one in the whole sky we can find.
So, again speaking just of odds, it's not too silly to think that there were unusual circumstances for this to have come about. Maybe, for example, two or three smaller black holes formed first, then collided and merged to form this much bigger one. Forming smaller ones through accretion of material is doable; recent research showed that it's possible to get really big ones early on, though just how big is hard to say.
I'm spitballing here. No matter what, J1342+0928 is both a record breaker and a thorny problem. Something about our ideas of what the Universe was like when it was in its infancy needs some adjustment.
But you wanna know a secret? That's awesome! Scientists love a good mystery; it's one of the biggest reasons most of us became scientists in the first place. Or, at least, it's one of the reasons scientists stay being scientists. Science isn't just memorizing stuff and adding to the pile of knowledge we already have; it's finding something that doesn't make sense and using the clues and evidence provided by nature to figure out what we've missed.
Science is one of the coolest things we humans do: It's making sense of the Universe itself. Even when that Universe doesn't seem to cooperate and abide by our previous understanding.
That's how we learn. And this time we learned something amazing. The cosmos knew how to make really big black holes when it was still a baby. Now we just have to figure out how.