Create a free profile to get unlimited access to exclusive videos, sweepstakes, and more!
The Universe is shaking: 39 new gravitational wave events added to the list
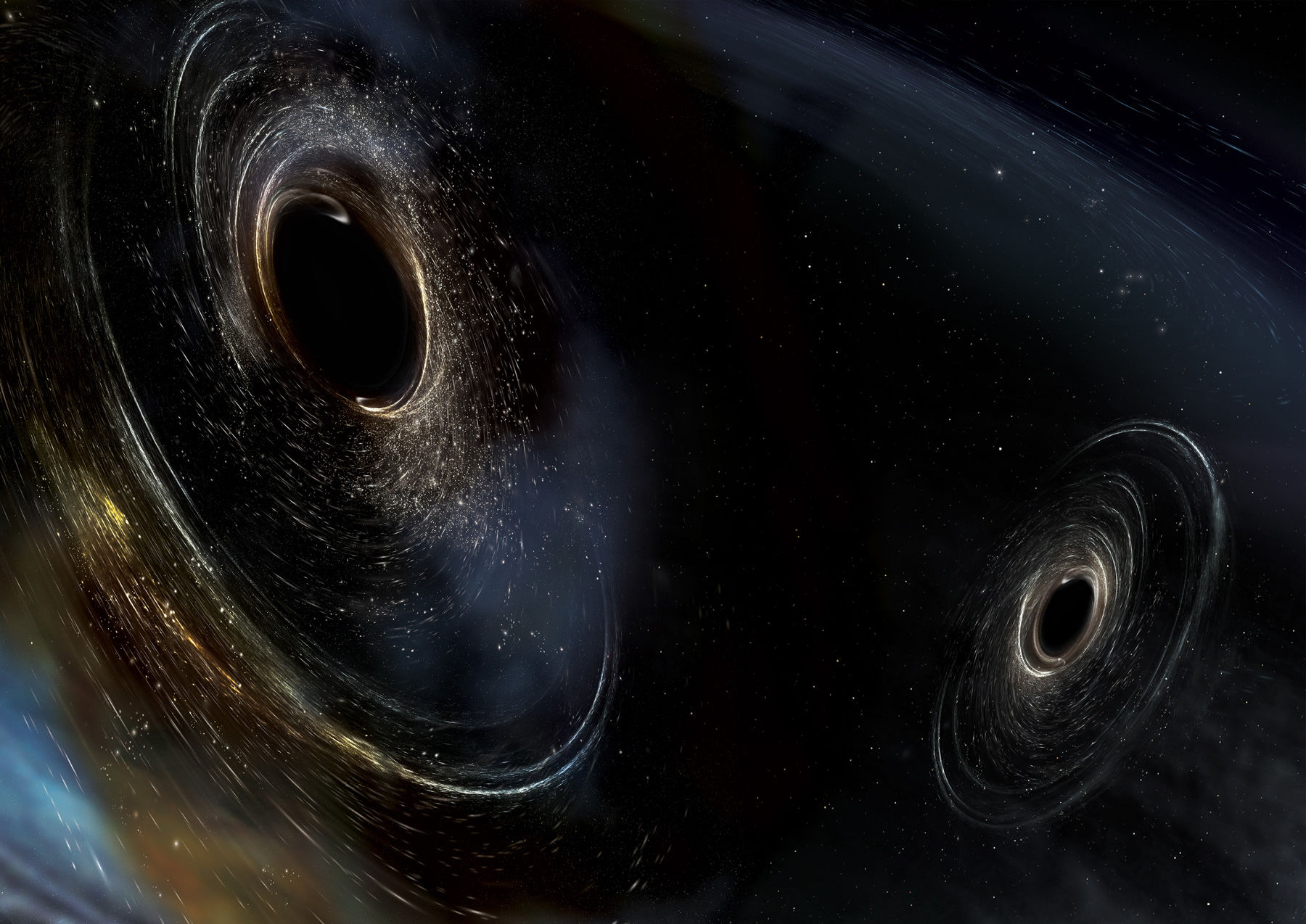
The Universe is positively vibrating with gravitational waves.
Scientists with the LIGO/Virgo collaboration have just announced they found a total of 39 gravitational wave events in the first six months of their third observing run, 26 of which were previously announced, but 13 of which are new. Given that the first two observing runs yielded a total of 11 combined, this is a huge leap. And it's only the first half of the run.
This is a big deal. Gravitational waves are literally ripples in the fabric of spacetime generated when extremely dense compact objects like black holes and neutron stars spiral together and merge. The merger event shakes spacetime, hard, generating thousands of times more energy than exploding stars, but it all goes into gravitational waves that move outward at the speed of light. They're ridiculously difficult to detect, and it took decades to build detectors sensitive enough to see them.
This new passel includes some oddballs, including the lowest mass confirmed black hole merger, and what may have been a neutron star torn apart and eaten by a black hole. It also means some cool science comes from having enough detections to start extrapolating trends.
LIGO, the Laser Interferometer Gravitational-Wave Observatory, was the first to detect them in 2015, created by a pair of massive black holes that ate each other, forming a single far more massive black hole. A few more detections followed, and over time upgrades to the observatory made it more sensitive and the rate increased. LIGO is an American facility (actually a pair of detectors, one in Louisiana and the other in Washington state) and was eventually joined by Virgo in Italy.
As gravitational waves pass through the Earth, they literally shrink and expand spacetime. The observatories are underground in long perpendicular tunnels. Mirrors are set up a few kilometers apart that split and reflect powerful lasers. The beams are then combined together, and if a gravitational wave passes through the facility the distance between the mirrors changes, affecting the laser light. When combined, they create an interference pattern, similar to when you slosh around in a bathtub making waves and they sometimes combine to make even bigger splashes.
Mind you, the amount the waves change the distance in LIGO is incredibly small: about 1/1000th the width of a proton! But that's enough to create a pattern in the laser light that can be seen.
The first two observing runs went well, finding 11 positively identified events, including black holes merging and even a pair of neutron stars colliding, exploding, and creating what may be a black hole. But before the third run the facilities were upgraded, including better lasers, better mirrors, and better techniques to reduce noise (a truck driving down a nearby highway is easily detectable by LIGO).
They also created better "waveforms." These are patterns made by the mergers in the detectors. As the two objects spiral together, the gravitational waves they send out get higher in both amplitude (strength) and frequency. This creates a pattern called a "chirp" (because a chirp, like a bird chirp, is what you hear when sound waves increase in frequency and volume), and they're different for different mergers. So, two small black holes merging will generate a different waveform than two much more massive ones, or a small one and a massive one. A library of theoretical waveforms is used (created using the equations of General Relativity) to match what's seen and determine what kind of event occurred.
The new mergers include a few exceptional cases. One of them, called GW190924_021846 (for Gravitational Wave event on 24 September 2019 and the UTC time) was from the merger of the two confirmed lowest mass black holes yet; one was 6 times the Sun's mass, and the other 9. A merger detected earlier may have had a lower mass component, but it's not clear that it was a black hole.
Another, GW190426_152155, may have been from a black hole tearing apart and eating a neutron star, but the signal was so faint it's hard for scientist to be sure.
Now that so many mergers have been seen, some interesting statistics are starting to emerge. For example, when looking at the two black holes that merge, there's a sharp dropoff in the number of mergers where the bigger of the pair is more massive than about 40 times the Sun's mass. There are certainly black holes more massive than that, but they appear to be more rare (or at least don't merge with other black holes as often). It's not clear why.
There's a lot of interest in finding lower mass black holes, too. These are created when massive stars explode, and their cores collapse to form black holes. The theoretical lower limit to such a black hole is about 3 times the Sun's mass. What they're finding, though, is a dropoff in the number of black holes with around 8 times the Sun's mass and lower. Lower mass ones exist but they're seen much more rarely in mergers. It's possible that the Universe tends to make black holes from more massive stars, so the black hole itself is a lot more massive than the lower limit.
Interestingly, one of the papers that came with this release looked for gravitational waves coming from gamma-ray bursts, huge explosions that happen when black holes are first born. None was seen, which is an important result. Perhaps they're all too far away, or make waves too faint to detect. Hopefully one will happen close enough (though not too close!) to get a good detection.
I love this! Observational gravitational wave astronomy is a brand new field (even younger than exoplanet astronomy), and even though the first merger was detected just three years ago this discipline has come a long way. There are black hole mergers occurring somewhere in the Universe all the time, generating a background hum of gravitational waves that, one day, we should be able to detect. By then we'll have thousands of mergers detected from all sorts of objects.
What bizarre and wondrous things will we have learned by then?