Create a free profile to get unlimited access to exclusive videos, sweepstakes, and more!
This AI isn’t just any artificial brain—it actually functions like the human brain
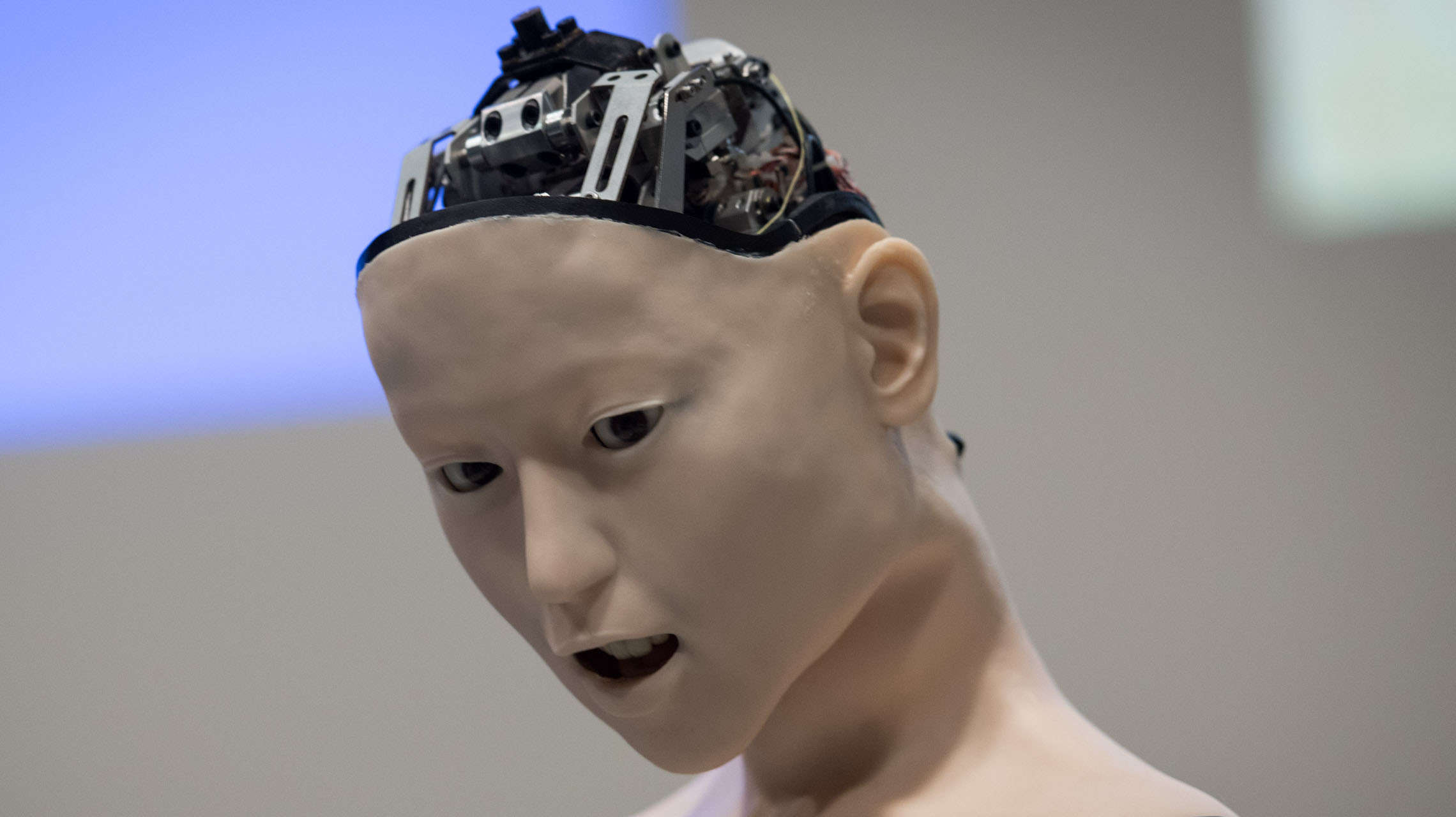
There is often a misconception that computers can beat the human brain at everything because they are programmed to know all the answers. The reality may not be what you think.
AI can often screw up because transistors don’t function like neurons. That is why scientists are trying to model it after the much more adaptable system of neurons in the human brain. Even the most advanced types of artificial intelligence can still glitch because they need to process and store information separately instead of taking on both at once. That might start to change now that researchers Xudong Ji and Jonathan Rivnay of the University of Hong Kong and Northwestern University are bridging the gap between man and machine.
“Our research focused on an organic electrochemical transistor (OECT), and we found that the operation of an OECT is very similar as a neural synapse,” Ji and Rivnay, who led a study recently published in Nature Communications, told SYFY WIRE. “A synapse relies on the action potential-driven transport of neurotransmitters, while an OECT relies on ions being transported in a similar way.”
Neurons use action potentials, or spikes in voltage, to talk to each other. Synapses are where neurons connect. Sort of like the moment you click send when texting someone, a neuron that wants to communicate with the one on the other side of the synapse will release a chemical known as a neurotransmitter, which carries information. Neurotransmitters will either excite neurons on the receiving end into also firing an action potential and passing on the message, or keep them from pressing that chemical send button. Inputs throughout the brain’s network of neurons determine whether an action potential is a go.
Even though both are technically electrical gizmos, neurons have an advantage over computers because of their synaptic plasticity. The strength between neuronal connections can be improved over time. Existing computer transistors are much more rigid. The closest we have gotten to some vague semblance of plasticity is something called a memristor (memory resistor), which can both process or “write” and remember or “read”, but things can get complicated. You have to separate the processing and memory functions if you want a memristor to remember things, and that can cause problems with integrating that memory into another system later on.
Never mind that memristors use up too much energy when things have to be switched around. They also aren’t biocompatible, so they reach a dead end when it comes to developing tech that can integrate with the human nervous system through a robotic limb or help people regain use of limbs that suffered nerve damage.
“Memristor drawbacks have brought researchers’ attention to synaptic transistors, especially organic electrochemical synaptic transistors whose write and read operations do not need to be decoupled,” Ji and Rivnay said. “In our device, the memory effect can be maintained with coupled write and read operation because of the novel ion-trapping active material. This enable more simplified circuit integration in the future.”
Like an actual brain, the device needed to be trained to prove that it is capable of functioning like a neuron network by exchanging ions instead of neurotransmitters. They taught it associative learning similar to the psychological experiment involving Pavlov’s dog. Instead of associating food with a bell to the point when the dog started salivating at the ring of a bell, the computer brain would learn to associate light with pressure. After a light was turned on, pressure would be applied, and the circuit’s pressure and light sensors would pick that up. It eventually learned to associate light with pressure even when pressure was not applied after the light appeared.
Further experimentation is still needed here. Anything that can lower the power consumption is a plus, and it needs to be tested with different materials to see what it can be used with. It already has the advantage of being made of soft organic polymers that are biocompatible, and it will have to be advanced further to be used in a variety of applications. Though Ji and Rivnay are excited about their technological breakthrough, it will take a while (not to mention much more complex training) before it starts showing up in everyday electronics and turning the real world into a sci-fi dreamscape.
“For now, the pressure and light sensors are separated from the neuromorphic circuit, so one of the further improvements is to integrate them all together in a flexible form,” they said. “Such a device can be easily and directly applied to smart wearable electronics, smart robotics and implantable devices that directly interface with living tissue— even the brain.”
Merging a biocompatible computer brain with a living brain might be the ultimate integration of living tissue and robotics. Neuralink better watch out.