Create a free profile to get unlimited access to exclusive videos, sweepstakes, and more!
Why do some impact craters have rays?
When you look at the full Moon through binoculars or a small telescope, one of the most prominent features on the surface is the crater Tycho. It's an impact feature about 86 kilometers wide, situated near the southern edge of the Moon's near side. It's relatively young — maybe 100 million years old — and fresh craters tend to be brighter, making it easy to spot.
But that's not why it's so prominent: It's the rays, the collection of long, bright features pointing radially away from the crater. Tycho sports rays hundreds of kilometers long, some over a thousand.
The rays form from plumes of material ejected during the impact, which then settle onto the surface. Now here's the funny thing: I always figured that their formation was well understood. I mean, these are incredibly obvious and well-documented features, not just on the Moon but on most crater-ridden worlds. Mercury has crater rays so long the planet looks like a watermelon!
So I was pretty dang surprised to learn we didn't know how they form. At least, not until recently. A new research paper outlines how impacts generate rays, and it's very cool. Even better: The scientists got the idea after watching YouTube videos of high school students doing the classic “make craters by dropping rocks into a box of flour” experiment!
Yes, seriously. These experiments are done in classrooms and science fairs all over the world. You take a wooden frame of some kind maybe a meter wide, pour in a layer of flour a few centimeters deep, then drop rocks on it from a height. The impact forms craters, just as you'd expect (sometimes you can put in a layer of cocoa powder to show what happens to stuff under the surface, too).
I've done this myself, many times. What the scientists noticed is that when the teacher resets the experiment, they smooth over the flour on top. I've always done that myself. And when that's the case, cratering impacts rarely leave rays.
But when students do the experiment, they sometimes leave the surface messy… and when they do, rays are more likely to form!
Whoa.
So the scientists took to the lab, recreating this experiment on a more sophisticated level. They used different sized balls to mimic asteroids, and varied the texture of the surface of the impact site. Sometimes it was smooth, and sometimes it had undulations in it, ripples. And when they did that, the impact made ray systems.
Not only that, they found a relationship between the number of prominent rays generated and the size of the ball compared to the distance between the ripples — the number of rays created in an impact scales with the size of the ball divided by the distance between the ripples (what they call the wavelength). So a big impactor hitting terrain with lots of narrow ripples makes more rays than a smaller ball would, or if that big one hit something with wider undulations. Watch:
So. Cool.
So this works with low-speed impacts, the kind you can do on a tabletop where you're really dropping rocks onto a surface. But what about hypervelocity impacts, more like real life, when an object is moving at a dozen kilometers per second or faster?
They simulated impacts like that, and found it still worked! The bigger the ratio between the impactor and the undulations, the more rays were made. They found that the physics is a tad complicated, but basically the undulations focus the shock wave generated by the impact — and it's that wave that accelerates and flings out the debris (called ejecta). The number of rays doesn't seem to care what speed the impactor had, just its size.
They also found that the material that forms the rays doesn't come from the crater itself, but from material on the surface around the impactor, specifically from a narrow ring around it.
Another interesting feature of this idea is that if they count the rays around an existing crater, and carefully measure the topography of the area around it, they can estimate the size of the impactor. For Tycho, they estimate the asteroid that carved out that gorgeous crater was about 7.3 kilometers across — not much smaller than the one that hit Earth 66 million years ago and ended the Cretaceous period, along with 75% of all species of life on Earth.
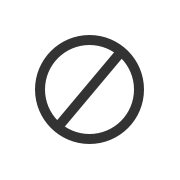
I have to say, I love everything about this! From the way they got the idea — watching student videos! — to recreating the event, to finding the pattern, and then using that to get the physics and turn this into an impact measurement tool… it's all wonderful. And a great story.
The full Moon is generally considered an irritant to observational astronomers: It's so bright it washes out faint objects. And if you like to observe the Moon itself, when it's full there are no shadows, so features like mountains and craters are harder to spot.
But in fact some craters really shine when the Moon is full, fresh young ones with brighter material inside and around them, ejecta not old enough to darken due to micrometerite impacts and solar radiation. Tycho, Aristarchus, Kepler, Copernicus… so many of these literally get their time in the Sun for us to marvel over them here on Earth, displaying their ray systems that reach so far across the surface.
And now we finally know why.