Create a free profile to get unlimited access to exclusive videos, sweepstakes, and more!
Another black hole first: Astronomers capture a ring of light as photons orbit M87A*
Eerie black hole photon ring observed for the first time.
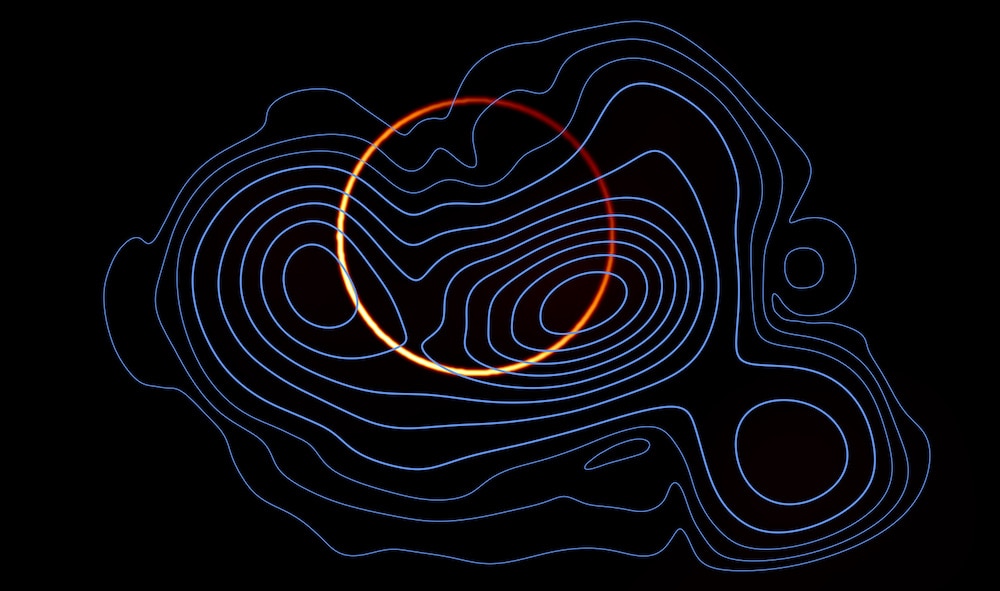
In 2019, astronomers revealed the first ever close-up image of material swirling around a black hole. It was a staggering achievement, combining the power of many radio telescopes across Earth to make a virtual one — called the Event Horizon Telescope — the size of our planet.
Follow-up work revealed the magnetic field in that accretion disk, as well as details on the jet of matter heavily accelerated and flung away at a decent fraction of the speed of light.
But there’s still more information to tease out of that data: A team of astronomers has just released an image showing the black hole’s photon ring, a narrow ring of light that literally comes from photons that orbit around the black hole before escaping into space [link to paper].
Black holes are super weird.
The black hole in question is M87* (“M 87 star”), a supermassive monster in the center of the equally huge nearby elliptical galaxy M87, which is about 55 million light-years from us. We’ve known about this black hole for decades; M87 is what’s called an active galaxy, where a vast amount of energy is blasted away from a very small region in its core. This is due to material falling in from the galaxy onto the black hole. As it does so it piles up outside the event horizon — the Point of No Return — in a flat disk called an accretion disk. The material gets incredibly hot due to friction, achieving temperatures of millions of degrees. Material this hot glows fiercely, including in high-energy X–rays, a sure sign a black hole is powering it.
The original M87* image shows material in the accretion disk roughly 40 billion kilometers across. It’s fuzzy, which isn’t hugely surprising given its vast distance from us. The ring is incredibly small on our sky, only 40 microarcseconds across. An arcsecond is an angular measure, where 3,600 of them make a degree; the full Moon is about 1,800 arcseconds across on our sky, or 45 million times bigger than M87*’s ring!
But there’s more to this. In the image we’re actually seeing the combination of two things. One is the material swirling around the black hole, which is extended; that is, not very sharp. The other is the photon ring, a sharp, narrow ring of light caused by the intense gravity of the black hole. As light passes near the event horizon its path is bent by the black hole’s gravitational pull. Because this is what a lens does this effect is called gravitational lensing, and not surprisingly it gets stronger the closer you get to a black hole.
Light coming in at the right angle gets bent so much it gets sent toward us, forming a fairly fuzzy ring called the n=0 ring. But light approaching the black hole that passes at just the right distance can actually orbit it once before escaping. This is called the n=1 ring, and is sharply defined. There are other rings where the photons go around twice or more times before heading out again, which have a somewhat smaller diameter than the n=1 ring; those photons have to approach the black hole more closely to be constrained enough to whip around it several times.
Theoretical models show that as much as 30% of the light in the original image comes from the n=1 ring. The problem is that this light is mixed in with the more extended light. How to separate them out?
The astronomers employed a method that used observations from the telescope, models of what the emission from a ring and accretion disk should look like using General Relativity — necessary due to the very strong gravitational region around the black hole — and the very complex math of how ultrahot material flows, called magnetohydrodynamics. This showed what the two combined looks like, and then they used Bayesian statistics to tease the two out from each other. That’s a kind of statistics that allows you to use prior knowledge of a system to predict how it will behave.
In the end, they were able to separate out the two signals, showing the narrow photon ring as well as the more extended emission. This is the first time this has ever been done. They even showed that the ring is stable and relatively unchanging over the seven days of observations, as expected, even while the extended accretion disk light was changing as material moved around the black hole.
And it’s not just a matter of idle curiosity. The size of the ring depends on the mass of the black hole. Working backwards, they find the mass of M87* is 7.13 ± 0.39 billion times the mass of the Sun. Earlier measurements found it to be lower, closer to 6.5 billion, but the team thinks the new number is more accurate, and in fact the biggest source of uncertainty now is in the distance to M87 itself.
Once they separated out the extended emission they saw something else that’s amazing: It’s consistent with what’s expected from the very base of the jet of material shooting outwards at tens of thousands of kilometers per second. Theory indicates the jet is focused by a complex process where the magnetic field of the material in the inner accretion disk extracts energy from the spin of the black hole, a source of immense power. This causes the jet itself to rotate, and the emission seen is consistent with the jet spinning clockwise from our view, which matches earlier results as well.
Mind you, all of this was in the original data, but it took this new statistical technique to pull it out and disentangle the sources.
The Event Horizon Telescope is the gift that keeps on giving. In 2022 it took similar images of Sgr A*, the supermassive black hole in the center of our Milky Way Galaxy. What treasures are in that data as well? And what will we find as we point this world-sized telescope at other black holes anchoring the centers of other galaxies?
These sorts of observations were theoretical not all so very long ago. Now they’re reality, and we’re learning even more about what happens just above the infinite dive into a hole in spacetime.