Create a free profile to get unlimited access to exclusive videos, sweepstakes, and more!
Event Horizon Telescope sees the magnetic engine behind a supermassive black hole's immense power
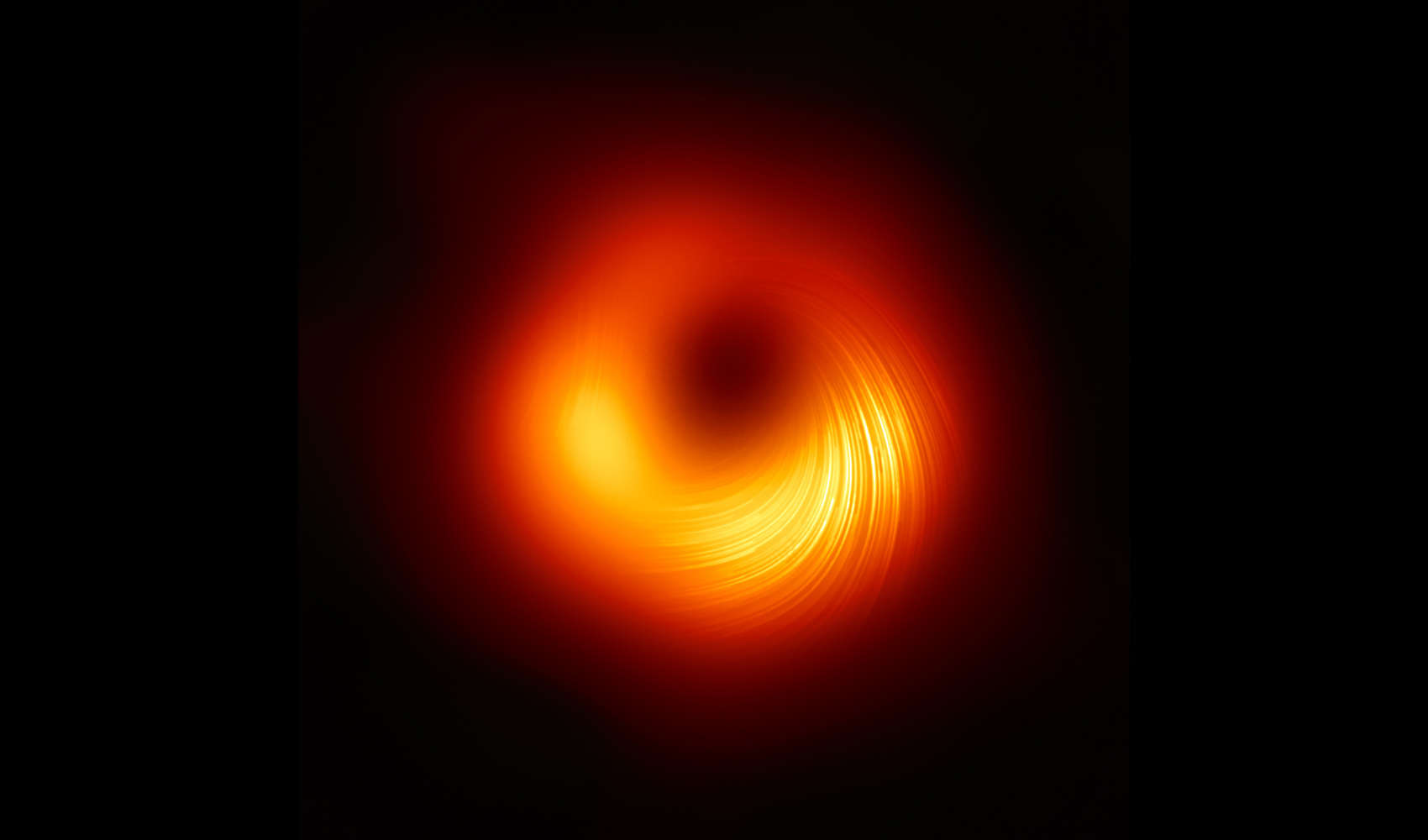
In 2019, astronomers stunned the world, releasing an epic image of material swirling around the supermassive black hole in the core of the galaxy M87 55 million light years from Earth. They connected radio telescopes from around the planet to create the Event Horizon Telescope, a virtual telescope mimicking the power of a telescope the size of our planet, to achieve this amazing observation.
More observations also showed the material around the black hole changed in brightness, even as the ring itself was fairly constant in shape and size. All of this helped astronomers understand what happens to material right before it takes The Final Plunge.
The glow of light from the swirling matter was seen in radio waves, far outside what our eyes see. Light like this isn't made because the matter is hot — though it is incredibly so — but because of fierce and fiercely complex magnetic fields embedded in that material. While the images were stunning, they didn't tell us much about that magnetic field, the force powering a substantial amount of the mechanics going on around the black hole.
Today, however, three papers have been published about just that. Using the Event Horizon Telescope data they were able to map the polarized light from the material, the key to investigating the magnetic fields themselves.
Light can be thought of a series of waves carrying energy away from an object. Normally, these waves are randomly oriented; if you imagine one like the string of a guitar, you can pluck it so it vibrates up and down, or left and right, or at any angle.
Some phenomena, however, send out waves that are aligned — like strumming a guitar gets the strings all vibrating horizontally. Light waves aligned in this way are said to be polarized. You may already be familiar with is concept: Light that reflects off metal or glass can be polarized. Polarized sunglasses have filters in them that contain molecules all aligned in one direction, such that when polarized light reflected off horizontal surfaces hits them it gets blocked. Only polarized light aligned with the molecules gets through.
Magnetic fields in a plasma (a gas that's had had one or more electrons stripped from its atoms) can cause polarized light to be emitted as well. The electrons spin around the magnetic field lines at nearly the speed of light, and when they do they emit light called synchrotron radiation. This light is polarized in the direction of the spin, and the strength of the polarization can be used to measure quite a bit of things about the plasma and the magnetic fields involved.
The material around the black hole forms a disk, and we see that as a ring of light around it (the disk on the far side of the black hole is visible because the intense gravity of the black hole bends it up and around toward us). The polarization of the light coming from the ring is parallel to the ring itself, indicating the magnetic field wraps around the ring from the black hole toward the outer edge (this kind of field is called poloidal which I find delightful).
Measuring this polarization indicates that the magnetic field near the black hole is quite strong, and actually helps keep the material in the disk at the inner edge, temporarily slowing some of it from falling in. They measured the rate at which stuff is falling in as 0.0003–0.002 of the Sun's mass every year. That may not sound like much, but in fact it means that at the top of that range the black hole is consuming as much as two Jupiters worth of material every year.
Or, if you prefer, it's eating the equivalent of the Earth twice a day.
<sudden panicked screaming while running around in circles>
That's phenomenal. They also found that the magnetic field strength is something like 1–30 Gauss, or roughly twice to 60 times stronger than Earth's. Again, that may not sound like much but it's acting over a vast amount of space, hundreds of billions of kilometers at least. The power of such a magnetic field is immense.
In fact, black holes like this can launch twin beams of matter and energy away from the disk, what astronomers call jets, and these are so strong they can stretch for hundreds of thousands of light years, far larger than entire galaxies. The energy of such jets is just mind-crushing; the kinetic energy of the material alone (its energy of motion, how much energy it took to get it up to speed) can be many of billions of times the energy the Sun emits!
We know these are also magnetic phenomena, caused by the twisting up of the inner magnetic field of the accretion disk into a tornado-like vortex, but it's not clear how that happens. By measuring the magnetic field properties so close to a black hole, astronomers will gain insight into these strange and terrifyingly powerful features. M87 has jets like this (we only see the one aimed more or less toward us due to relativistic effects), and it was studied in the new analysis as well.
Measuring the polarization of the light is an incredibly difficult and painstaking process, which is why these observations are being released only now; it took much longer to analyze the data than to make the image we saw two years ago. But the information stored in such data is worth the effort and the wait.
And to me the actual numbers and mechanics of this particular black hole, as tremendously cool as they are, aren't the real treasure here. What's really valuable is the knowledge that we can actually do this. It's hard, and it's groundbreaking, but it can be done. There are a lot more black holes out there — including Sgr A*, the 4-million-solar-mass behemoth in the center of the Milky Way — that can be studied in this way. We may need bigger and more powerful radio telescopes, and we may need more computer processing power, but these observations show that this is a technical issue, an engineering problem to solve, not a physical one.
We can peer at matter millions of light years away, sift the results for specific kinds of light, and use that information to better understand what happens when a black hole 6.5 billion times the mass of the Sun rips a hole in the fabric of reality. I'd say that's worth knowing.