Create a free profile to get unlimited access to exclusive videos, sweepstakes, and more!
Record breaker: Astronomers find the most massive neutron star known. Probably the most massive one ever.
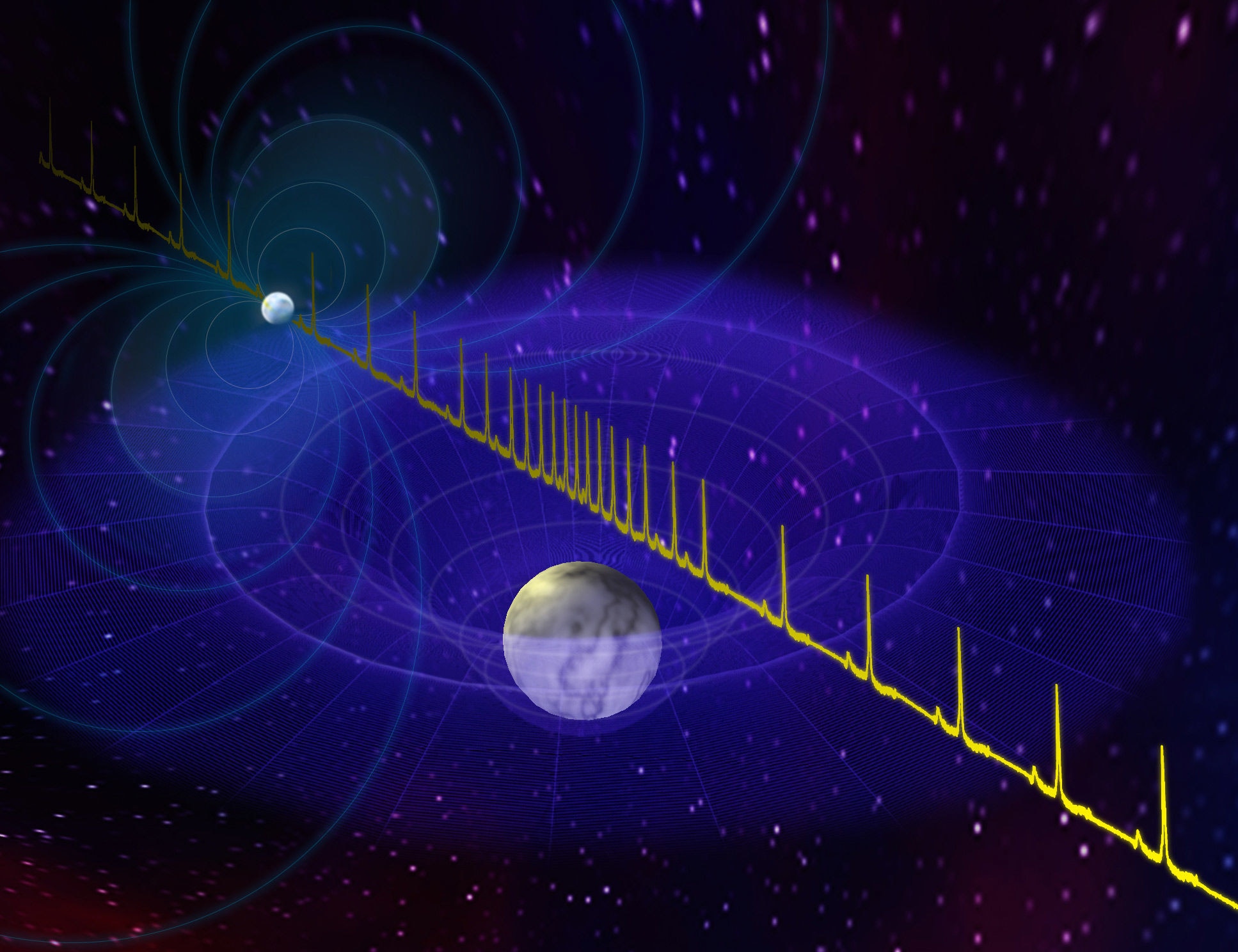
A team of astronomers has made an important observation that has helped significantly narrow down a question that's been nagging at scientists for years: How big can a neutron star get?
I won't keep you in suspense… but the price for getting the answer early is understanding it, so you have to promise to read the rest of this article, too. OK?
OK. They measured a neutron star that appears to be the most massive ever seen: 2.14 (±0.10) times the Sun's mass. That is a whopping big neutron star.
Or I should say a little one: Even though it's more than twice the mass of our Sun, it's only 30 kilometers across!
The neutron star is called PSR J0740+6620 (PSR stands for pulsar, and the rest is its coordinates on the sky). This is no ordinary neutron star, either. It's a millisecond pulsar, meaning it spins incredibly rapidly, very roughly about 346.531996493212 times per second.
Haha, "very roughly," I kill me. But that accuracy is correct! It has a powerful magnetic field, and as it spins it sends out blips of radio energy we can detect on Earth. These pulses — hence the term pulsar — are very, very stable in time, so the rotation speed can be measured with ridiculous accuracy.
But it gets better. The pulsar is part of a binary system, with a white dwarf companion, and they orbit each other every 4.7669446191 days (and yes, that number is known to that accuracy as well, and so it's just fun to write out). And even better than that, the orbital plane of the two is seen edge-on from Earth. Even though the white dwarf is only a few thousand kilometers across, it's big enough that the neutron star goes behind it once per orbit. When it does that, we get an eclipse of the neutron star.
That's critical! The white dwarf is the remains of a dead star that was once like the Sun (see below), with a lot of mass crunched down into a ball the size of Earth. It has ferocious gravity… not as fierce as the neutron star's, but enough to literally bend space around it in a measurable way (using Einstein's equations of relativity). Every time the neutron star passes behind it, the warping of space by the white dwarf's gravity delays the pulses from the neutron star. This delay is teeny tiny — just 10 microseconds. But the neutron's stars pulses are so regular that this delay can be measured!
The beauty of this is that the delay depends on the mass of the white dwarf. But the orbital period (which, remember, is measured to incredible accuracy) depends on the sum of the masses of both stars. Once they measured the delay, they got the mass of the white dwarf, which gave them the mass of the neutron star: 2.14 times the Sun's mass.
The white dwarf, by the way, is only (only!) about 0.258 times the Sun's mass. That's a little on the lower side of average, but not too surprising.
So why is this a big deal? It's because if you pile mass onto a neutron star, it'll collapse into a black hole. Knowing how massive they can get before pulling themselves down into an infinite hole is useful in understanding black holes.
Moreover, neutron stars are the densest "normal" objects in the Universe, and matter behaves very oddly when it gets that dense. It's impossible to replicate in labs, so we have to let nature be our lab. That in turn tells us a lot more about what goes on inside neutrons stars. Let's step back a bit further for a moment…
Like charges repel, and so negatively charged electrons repel each other (this is called electrostatic repulsion). But there's another repulsion they can undergo, too. If you squeeze normal matter really, really hard (like applying the kind of pressure you see in the core of the Sun), a quantum mechanical effect kicks in called electron degeneracy pressure. It's complicated (duh, it's quantum mechanics), but if you squeeze electrons together that hard the electrons repel each other due to this pressure, and it's far stronger than the usual electrostatic repulsion. When the Sun uses up all the available hydrogen in its core, the helium that's created will become so dense it will undergo this kind of pressure, and we say the core is degenerate.
This pressure is so strong it can keep the core of a star intact even if the mass of that core is up to 1.4 times the mass of the Sun. Above that, though, electron degeneracy pressure fails. It's not strong enough to support the core, and the core collapses. It'll shrink from the size of the Earth down to a ball just a couple of dozen kilometers across. At that point, another QM effect kicks in: neutron degeneracy pressure.
This is similar to electron degeneracy pressure but it happens between neutrons. It's a much stronger effect, though, and can support masses up to a little more than twice the Sun's mass. Above that, the core will collapse again and form a black hole.
But what exactly is that number? Calculations made using theoretical considerations get an upper limit of about 2.16 times the Sun's mass. Interestingly, the neutron star binary merger detected by LIGO-Virgo in 2017 allows another way to get that upper limit, and they found it to be… 2.17 solar masses. Huh. Pretty close.
So these observations match theory pretty well, and that's a good sign. This also makes PSR J0740+6620 the most massive neutron star known. If the numbers are correct, it's the most massive one we'll ever find. Perhaps though one even heftier will eventually be found, and astronomers will have to go back to their equations and figure that one out. But for now, it's a record breaker.
One last thing: The observations were done using the mammoth 100-meter Green Bank Telescope in West Virginia. It was part of observations made with NANOGrav, a project that looks at lots of pulsars in the galaxy to measure their pulse timing. The idea is that if somewhere in the Universe two black holes merge, they'll send out ripples in space called gravitational waves. As these waves pass through the pulsars it'll change the timing of the pulses. If enough pulses from enough neutron stars are measured, it may be possible to detect gravitational waves this way. It's a cool idea, and I really hope it pans out (follow astronomer Chiara Mingerelli for more info on this).
I don't usually write about cosmic records getting broken, because in general they're incremental. Like the most distant so-and-so ever seen, things like that. But this is actually a critical and even fundamental record; if a neutron star gets more massive than this, then it'll wave bye-bye to the Universe. And this research helps scientists probe the interiors of neutron stars, at least theoretically, giving them insight on one of the Universe's most bizarre objects.
[Correction (Sep. 18, 2019 at 00:15 UTC): Due to reading an old version of the paper instead of the final published one, I originally wrote the neutron star's mass was 2.17, when it is 2.14 times the Sun's mass. My apologies for my mistake! Lesson learned; make sure to check the final version before writing.]