Create a free profile to get unlimited access to exclusive videos, sweepstakes, and more!
A second wind – literally – for a scorching hot super-Earth
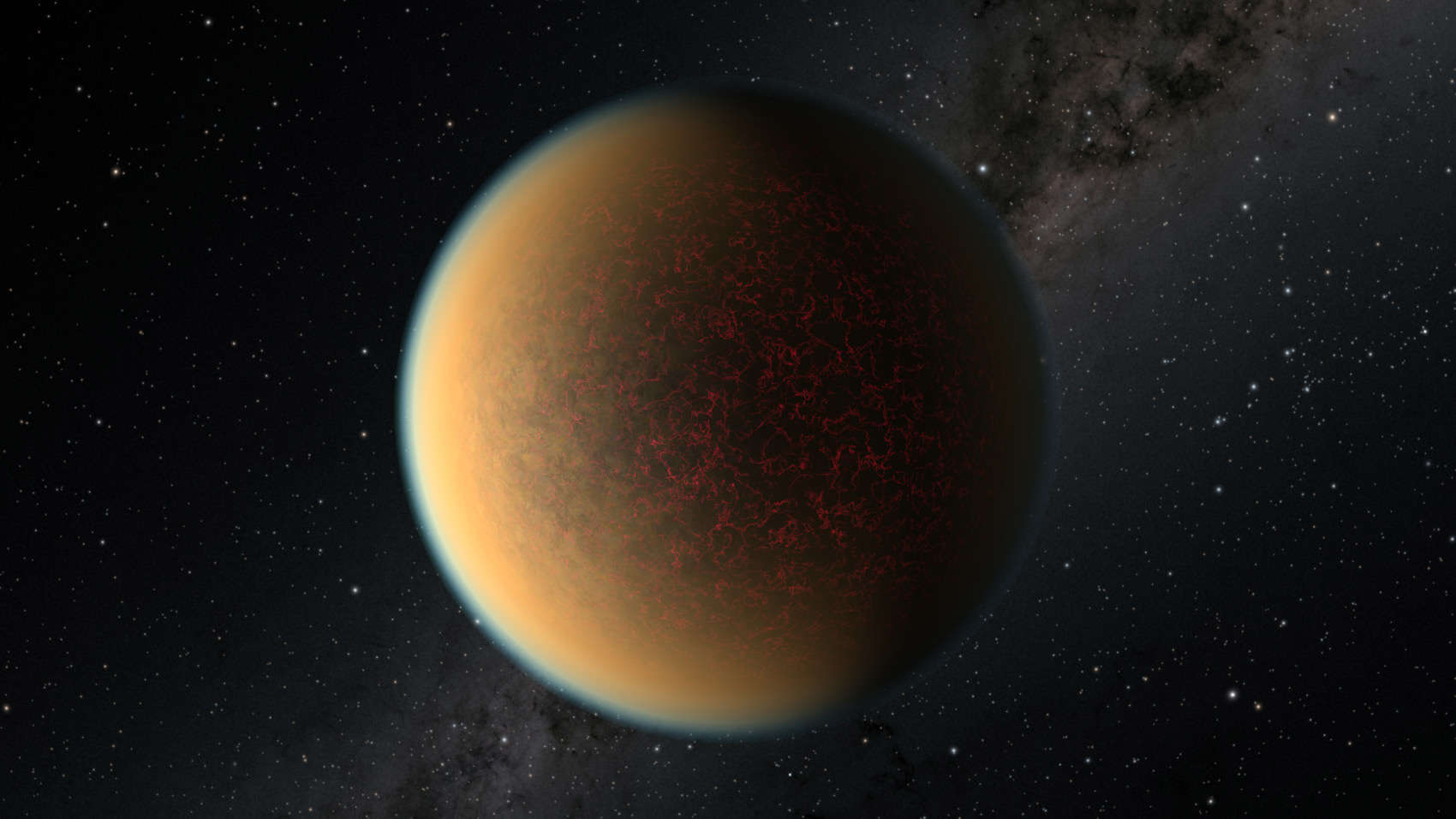
A nearby rocky planet orbiting very close to its host star may be sporting a second-generation atmosphere: It used to be more like Neptune, but the star blew the planet’s air away, and what we see now are noxious gases released by a magma ocean afterwards.
That’s pretty weird. But it’s also expected.
Planets form from a disk of material orbiting a young star. Farther out, where it’s cooler, are lighter atoms and molecules like hydrogen, helium, and ices; while closer in it’s too hot for those materials. Planets that form farther out tend to become gas giants, growing large and drawing in thick atmospheres.
But we also see gas giants orbiting their stars very close in. We think those form farther out, but friction with the material in the disk drops them toward their star. At some point the material thins out and the planet stops its inward migration.
Sometimes, though, a planet gets really close to its star. If that happens, the intense light and heat can literally blow the atmosphere away, a process called photoevaporation. This works best for smaller planets that have less gravity to hold on to the gases. A planet around the mass of Neptune or a little less (called “mini-Neptunes” because why not) is highly susceptible to this process. What’s left behind is the rocky-metallic core of the once-larger planet, usually 1 – 2 times bigger than Earth and up to 5 or so times its mass. A “super-Earth.”
But it’s also thought that while it’s forming, that rocky core can absorb lots of gas from the atmosphere, dissolving them into the magma. If the planet remains molten after the initial atmosphere is lost, the gases are then released, forming a second atmosphere.
To see if this can really happen, astronomers pointed Hubble at GJ 1132, a dim red dwarf star about 40 light years from the Sun. It’s known to host a planet, GJ 1132b, that’s about 1.7 times Earth’s mass and 1.16 times its diameter. That gives it a density of about 6.3 grams per cubic centimeter, a bit denser than Earth’s 5.5. That means it’s likely metal and rock like Earth, too. It orbits the star once every 1.6 Earth days at a distance of just 2.3 million kilometers, making it scorching hot: probably around 250° C.
… and that makes it a perfect candidate for the search.
GJ 1132b is a transiting exoplanet, meaning it passes directly in front of its star as seen from Earth. If it has an atmosphere, then light from the star will pass through it on its way here. When light passes through a gas, atoms and molecules in the gas absorb light at very specific wavelengths (colors), allowing astronomers to identify those materials.
They used Hubble to take a spectrum of the star when the planet was in transit, spreading the light out into individual colors. What they found is intriguing.
There are features in the spectrum that indicate the presence of various materials, including diatomic hydrogen gas (two hydrogen atoms bound together), hydrogen cyanide, methane, traces of acetylene, and haze (teeny particles suspended in the atmosphere). These were identified using models of planetary atmospheres to see what fit their observed spectrum best. The data are noisy — this is a difficult observation to make — but the model fits they obtained look pretty good.
This mix is what you’d expect from a planet that started off life as a mini-Neptune, got blow-torched by its star, lost its atmosphere, then slowly leaked out a new one (in a process called outgassing, a wonderful term I use in life for other obvious events) from molten rock.
This implies the planet is mostly molten, which is actually an issue. It’s not getting enough heat from the star to melt rock! But there’s a solution: The planet’s orbit is elliptical, so it gets closer to and farther from the star as it orbits. The tides from the star squeeze it harder when it’s close, and relent when its farther out. This periodic strain on the planet heats it up — we see a similar effect in moons of planets in our own solar system; for example Saturn heats up Enceladus that way, melting the interior of the tiny moon and giving it a liquid water ocean over its frozen surface, and with Jupiter’s moon Io, which is covered in volcanoes due to its internal tidal heating.
That’s why the planet is so hot, and could still be molten. In fact there’s a second planet orbiting farther out that is likely contributing to the process as well.
This is an impressive set of observations and analysis, and the results are fairly convincing, though I wouldn’t call it a slam-dunk. Like I said, these are difficult observations to make and there is some room here for uncertainty. The good news is that it looks like this method can yield results with more and/or deeper observations on Gliese 1132b as well as other candidate cooked former mini-Neptunes.
When I was growing up we thought planets (and we only knew of the ones in our solar system back then) pretty much formed the way they are and where they are. Hoo boy, were we wrong! There’s an incredible variety of planets, and that rich variation comes from what they’re made of, how they form, where they form, and, know we know, what happens after they form.
That’s why science doesn’t hold onto ideas too tightly. We allow for better knowledge and new ideas. If you don’t, look at the amazing stuff you’ll miss out on!