Create a free profile to get unlimited access to exclusive videos, sweepstakes, and more!
The Crab Nebula hulks out, sends *incredibly* high-energy gamma rays at Earth
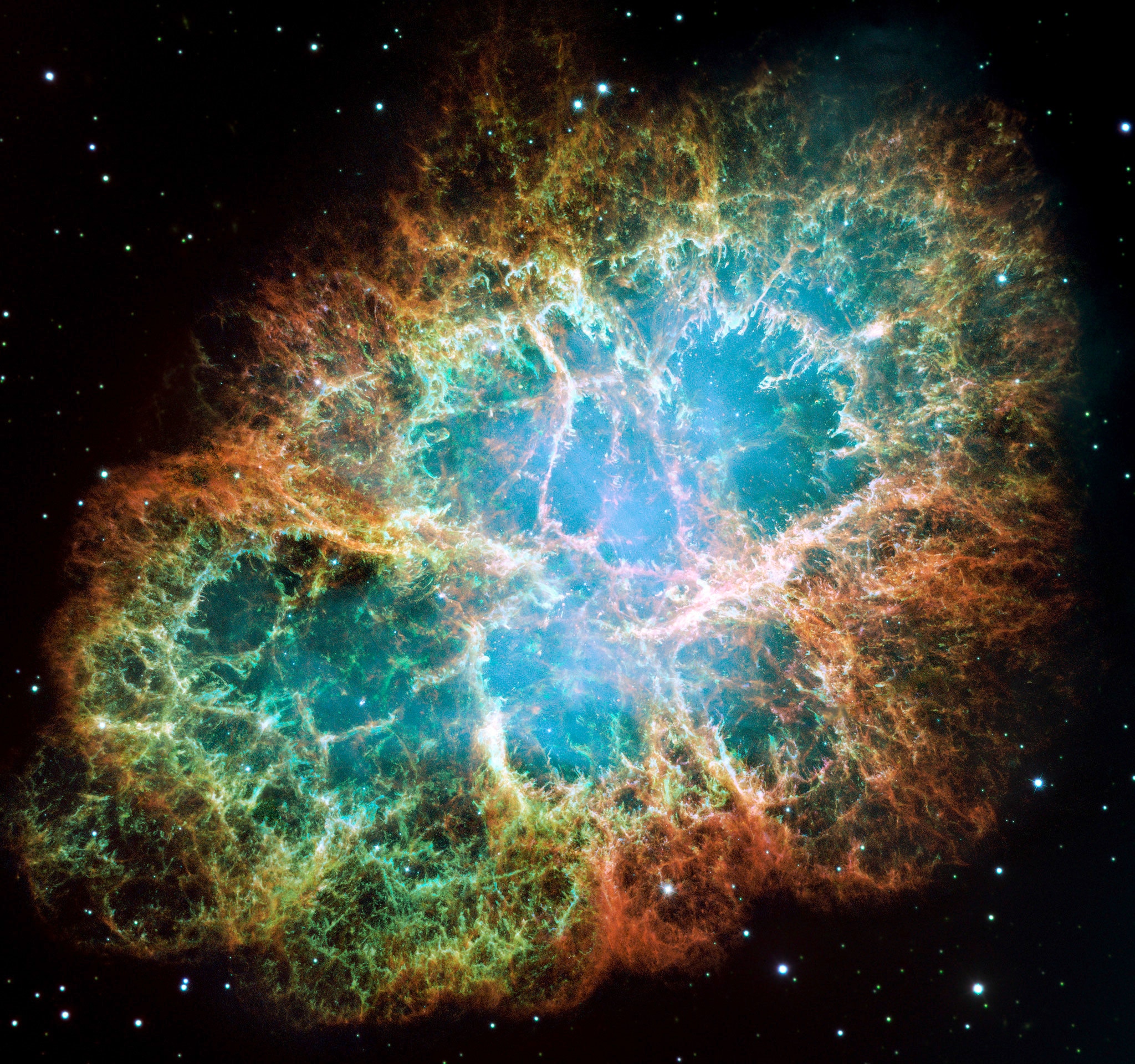
OK, I promise I'll explain all this, but I have to get this out of my system first: Astronomers have found that the Crab Nebula pulsar is blasting out extraordinarily high-energy photons by watching optical shock waves in water generated by faster-than-light muons.
I know, right? Just writing that down was cool.
Here's the deal. 6,500 light years from Earth is the Crab Nebula, the expanding gaseous and dusty debris from a massive star that exploded. The light from that event reached us in 1054, and because it's so close to us, relatively speaking, it's been studied extensively ever since the nebula was discovered. It's fair to call it one of the most studied objects in the sky.
Yet it can still surprise us.
In the center of the gas cloud is a neutron star, the extremely dense remains of the core of the exploded star. When the star exploded the core collapsed, cramming a couple of times the Sun's mass worth of matter into a ball just 20 kilometers across (for comparison, the Sun is nearly 1.5 million km wide). The material making it up, called neutronium, is so dense that it's just on this side of being a black hole.
It also spins rapidly and has a fierce magnetic field. Subatomic particles are accelerated to high speeds and blast away from the poles of the star — and in most cases the rotational axis and magnetic field axis don't align. So you get these beams sweeping around as the star spins, and if a beam passes over us we see a blip of light, pulsing every rotation. That's why we call these objects pulsars.
Pulsars, including the Crab pulsar, have been detected across the electromagnetic spectrum, from radio waves to high-energy gamma rays. And that's where things get interesting. At extremely high energies, gamma-rays are in a sense unstable. They can undergo what's called pair production, creating matter from their energy. When they do, they make a particle (like an electron) and its antiparticle (like an anti-electron, called a positron). The higher energy they are the more likely this is to happen, so it's thought that there is an upper limit to what we see coming from the Crab pulsar; over that distance a super-high-energy gamma ray isn't likely to make it all the way here.
There’s a lot of interest in figuring out what that limit is. The physics is somewhat daunting, but the measurement of such energetic photons can tell us a lot about the environment around the pulsar.
Detecting them, though, is difficult. But nature provides us with a trick. When such a photon slams into our atmosphere, it deposits a lot of energy into the air. This creates a huge wave of subatomic particles such as muons that then spray out from the collision like shrapnel. Here's the fun part: The speed of light in a vacuum is the ultimate speed limit; nothing can move faster. But due to some more complicated physics, the speed of light is lower in some media like air and water. It's possible for particles moving near the speed of light in a vacuum to actually be moving faster than light can in that medium! When they do, they create something that acts like a sonic boom, except it's made of light, not sound. This characteristic blue glow is called Cherenkov radiation.
The beauty of all this is that these flashes of light can be traced backwards to get the direction to their source in the sky. If you take a tank full of water and line it with detectors, this can be done with a lot of accuracy. You can also figure out the total energy of the photon that created the cascade in the first place.
The energy unit for photons usually used is called an electron Volt (or eV). A visible light photon, the kind we see, has an energy of very roughly 2 eV. X-rays are in the thousands of eV (or keVs) and gamma rays in the millions (MeV) or billions (GeV).
Astronomers using the Tibet Air Shower-Gamma Experiment have been using it to look for gamma rays from the Crab pulsar. They released their results in a new paper, and when I read it, well, the hair on the back of my neck stood up.
They detected fully two dozen gamma ray photons with energies greater than 100 trillion eV. That's incredible. These are the highest energy photons ever detected from an astrophysical source, and I imagine astronomers will have an interesting time figuring out how they made it all the way from the Crab to here intact.
Now, yeah, I know that's a weird unit and if you're not familiar with it you're probably just kinda nodding your head and thinking "OK." But to an astronomer, that's a phenomenally huge amount of energy for a single photon. The highest energy gamma ray they detected had an energy of about 400 TeV. It would take about two hundred trillion visible-light photons to equal that much energy.
Yeah, I know. Still too weird. OK, let's try this. A photon is basically a bundle of energy. It's incredibly tiny, submicroscopic, literally quantum mechanical in scale. But the energy it has can be described in our macroscopic world.
When something is moving, it takes energy to get it up to speed. This energy of motion is called kinetic energy, and it depends on the mass of the object and its velocity.
If we consider these hugely energetic photons from the Crab pulsar, what would be their macroscopic equivalent of the energy of a moving object?
Well. A common housefly has a mass of about 10 milligrams (or 1/100th of a gram). A typical flying velocity for one is about one meter per second. That gives it a kinetic energy of about 50 ergs. Doing the conversion, that means that one of those energetic photons from the Crab Nebula has an energy more than 10 times that of a housefly in flight.
Have you ever been minding your own business when a housefly buzzing around bumbles into you? It's irritating, isn't it, because you can feel it even through your clothes. It's unsettling.
Well, getting hit by something with ten times as much energy would be pretty obvious. Do you see where I'm going with this?
To phrase this a little differently:
An object just a few kilometers across — but made of material so dense that each sugar-cube sized chunk outweighs all the cars in the US — generates power on such an enormous scale that a single photon screaming away from it at 300,000 kilometers per second can travel 6.5 quadrillion kilometers across space with so much energy in it that if it were to hit you and you absorbed that energy in your skin you could actually feel it. From a single photon.
So, yeah.
The big question now scientifically is just how the Crab makes these fierce bundles of energy, and how they make it here. Now that we know such photons exist, and we can detect them, it'll help a lot to see more. Then we can see many of each is made at the different energies we see, and that'll give insight in the mechanisms behind this astonishing production.
Like I said, the Crab is studied so well it's amazing all by itself that it can surprise us. But when you're talking about young pulsars, well, they do pack quite a few of those.
[Correction note: I originally wrote that a visible light photon is about 0.5 eV, when it's actually 2. There's a range of energies from red to blue, but this is about in the middle.]