Create a free profile to get unlimited access to exclusive videos, sweepstakes, and more!
How big is a neutron star?
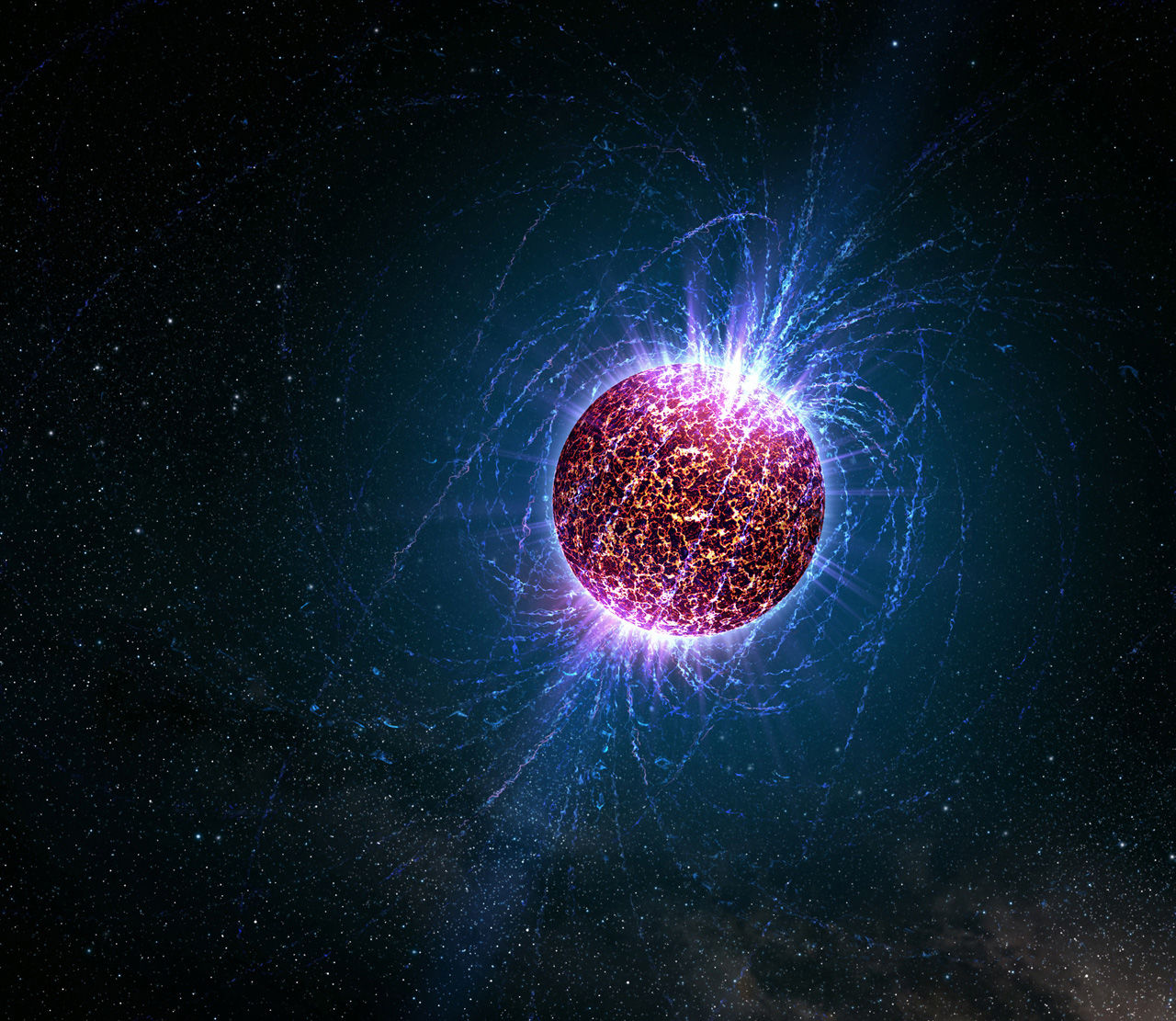
Neutron stars are the remains of massive stars after they go supernova; while the outer layers of the star explode outward creating fireworks literally on a cosmic scale, the core of the star collapses, becoming incredibly compressed. If the core has enough mass it'll become a black hole, but if it's shy of that limit it’ll become an ultra-dense ball made up mostly of neutrons.
The stats for neutron stars are sobering. They have a mass of up to over twice the Sun, but the density of an atomic nucleus: Over 100 trillion grams per cubic centimeter. That's hard to grasp, but think of it this way: If you compressed every single car in the United States into neutron-star-stuff, you’d get a cube 1 centimeter on a side. The size of a sugar cube, or a six-sided die. All of humanity compressed into such a state would be less than twice that width.
Neutron stars have a surface gravity hundreds of billions times Earth's, and magnetic fields even stronger. A neutron star half the galaxy away from us had a seismic event on it that physically affected us here on Earth, 50,000 light years distant.
Everything about neutron stars is terrifying. But for all that, we're still not exactly sure how big they are.
I mean, we have a rough idea, but the exact number is difficult to determine. They're too small to see directly, so we have to infer their size from other observations, and those are plagued with uncertainties. Their size also depends on their mass. But using observations of X-rays and other emission from neutron stars, astronomers have found they have a diameter of 20–30 kilometers. That's tiny, for such a huge mass! But it's also an irritatingly large range. Can we do better?
Yes! A group of scientists have approached the problem in a different way, and have been able to narrow down the size of these fierce but wee beasts: They found that, for a neutron star with a mass of 1.4 times the Sun (about average for such things), it will have a diameter of 22.0 kilometers (with an uncertainty of +0.9/-0.6 km). They find their calculation is a factor of two more accurate than any others done before.
That's… small. Like, really small. I'd consider 22 km a short bike ride, though to be fair doing it on a neutron star would be difficult.
So how did they get this number? The physics they employed is actually fiendishly complicated, but what they did in effect was solve a neutron star's equation of state — the physical equations that relate characteristics of an object like pressure, volume, and temperature — to get what the conditions would be like for a model neutron star with the mass fixed at 1.4 times that of the Sun.
They then used those results and compared them against observations of an event from 2017: A merger of two neutron stars that resulted in a colossal explosion called a kilonova. This event, called GW170817, was a huge watershed moment for astronomy, because the colliding neutron stars emitted powerful gravitational waves, literally shaking the fabric of the Universe. This was our first alert to the event, but then a large fraction of telescopes on and above the Earth aimed at the part of the sky where the merger was found to be, and saw the explosion itself, the kilonova. It was the first time an event was seen emitting electromagnetic energy (that is, light) that was first seen in gravitational waves.
It also put a lot of constraints on the neutron stars that collided. For example, after they merged they emitted light in a specific way, and it turns out that was inconsistent with the merged remnant having enough mass to collapse directly into a black hole. That happens around 2.4 times the Sun's mass, so we know the two stars together had less mass then that. Conversely, the light was inconsistent with the remnant being a neutron star well below that limit, too. It looks like a "hypermassive" neutron star was formed near that limit, lasted for a very short time, and then collapsed into a black hole.
All of this data was fodder for the scientists calculating the neutron star size. By comparing their models with the data from GW170817, they were able to greatly reduce the range of sizes that made sense, zeroing in on the 22 km diameter.
This size has interesting implications. For example, one thing the gravitational wave scientists are hoping to see is the merger of a black hole and a neutron star. This will definitely be detectable, but the question is will it emit any light that more traditional telescopes can see? That happens when material from the neutron star gets ejected during the merger, generating a lot of light.
The scientists in this new work ran the numbers, and found that for a neutron star of 1.4 solar masses and 22 km diameter, any black hole bigger than about 3.4 times the mass of the Sun would not eject any material! That's a very low mass for a black hole, and it's very unlikely we'd see any that low mass, especially one with a neutron star it can eat. So they predict this event will only be seen in gravitational waves and not light. On the other hand, that’s only for non-spinning black holes, and in reality most will have a rapid spin; it's unclear what would happen there, but I imagine a lot of folks will be running their models again to see what they can predict.
Having the size of a neutron star means being able to better understand what happens as they spin, as their ridiculously powerful magnetic fields affect material around them, how they accrete new material, and what happens near the mass limit between a neutron star and a black hole. Even better, as the LIGO/Virgo gravitational wave observatory folks fine-tune their equipment they expect their sensitivity to increase, allowing better observations of neutron star mergers, which can then be used to tighten the size constraints even more.
I've been fascinated by neutron stars my whole life, and to be honest that's the correct attitude. They're leftovers from supernovae; they collide and make gold, platinum, barium, and strontium; they are the powerhouse behind pulsars; they can generate mind-crushing blasts of energy; and are the densest objects you can still consider to be in the Universe (the physical object inside a black hole's event horizon is forever beyond our reach). I mean, c'mon. They're amazing.
And that about sizes them up.