Create a free profile to get unlimited access to exclusive videos, sweepstakes, and more!
Scientists read worms' minds to see what Spice they’re smelling
What's that smell?
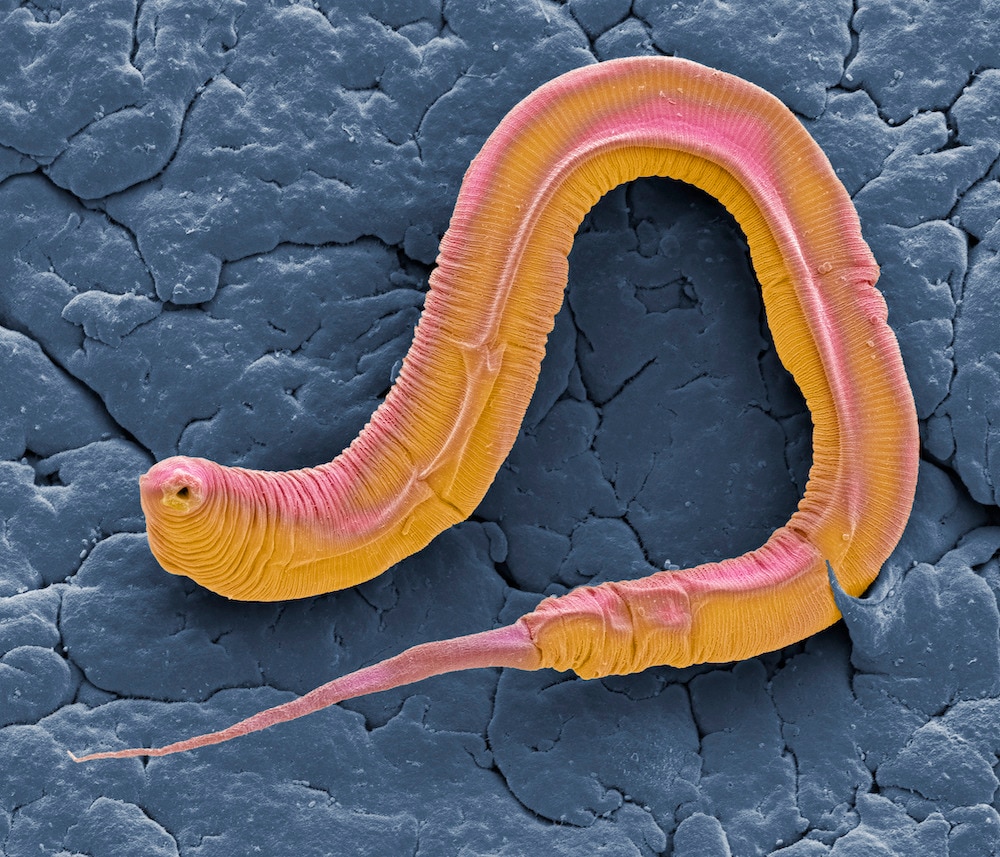
When faced with an overwhelming problem, one useful strategy is to break it down into more manageable parts. That’s the overarching philosophy that scientists are using to understand the way the human mind works.
Human brains have billions of cells all working in concert. Understanding the relationships that result in cognition are difficult, but there are other animals with much simpler neural systems which can act as model organisms to help pave the way.
The entire nervous system of Caenorhabditis elegans — a type of flatworm — consists of only 302 neurons and is comparatively well understood. Scientists have a fairly robust roadmap in terms of where those neurons are and how they’re connected with one another, making it easier to identify specific processes and activities going on inside their tiny minds. As a result, C. elegans is often used as a model organism for neuroscience.
In a new paper, scientists at the University of California set out to distinguish the neural patterns present when C. elegans was presented with certain stimuli. They succeeded in observing the activity and, more impressively, developed a system for predicting which stimulus was present, based on the neural activity alone. In short, they figured out a way to read the worms’ minds, at least under certain circumstances. Their findings were published in the journal PLOS Computational Biology.
The worms used in the study are transparent, allowing ready access to the inner workings of their heads. Researchers further illuminated the neural activity by engineering the neurons with a fluorescent marker which lit up when activated. Animals were then immobilized before being presented with olfactory stimulation. Scientists then focused on more than 50 of the 302 total neurons to observe what they were doing under different circumstances.
Each of the immobilized worms underwent three 21-minute-long imaging sessions during which they were either left alone to serve as a control, were presented with a buffer, or were presented with one of ten chemical stimulant scenarios.
Those scenarios included five different stimulants — benzaldehyde, diacetyl, isoamyl alcohol, 2-nonanone, and sodium chloride — at two different concentrations. In C. elegans, smells are first filtered by sensory neurons which organize them into group types including pheromones, potential food, and dangerous smells like acids or toxic compounds, among others. From there, the smells light up a network of activity and it’s these network properties that scientists set out to understand.
In each of the stimulant scenarios, 50 to 60 neurons activated, and researchers couldn’t readily tell the difference between them, despite knowing from previous experiments that the worms are capable of differentiating these smells. The nervous system was clearly doing something different for each smell, but initial observations of the neurons couldn’t decode what that was. That’s when the team turned to a mathematical process known as graph theory in hopes of gaining a clearer picture.
Using graph theory allowed scientists to not only see which neurons were firing, but how those neurons interacted with one another over time. And that was the key, specifically in the way the worms process salt stimuli.
This new view revealed a difference in the way smelling salt is processed in the brain when compared with other smells. The team captured those neural patterns, fed them into a machine-learning algorithm, and found it was able to predict the presence of some stimuli from the neural patterns alone.
The algorithm was also able to distinguish one of the other stimulants, while struggling to differentiate between the remaining three. This research highlights the complexities of neural activity, even in something as simple as a flatworm. And while teaching a computer to read a worm’s mind is cool all on its own, it’s not the endgame of this research.
Ultimately, scientists want to understand the way our own brains work, and these results suggest that at least some stimuli are decodable based on their neural properties. Unlocking patterns present under the influence of certain stimuli could give us a roadmap to understanding brain activity in more complex animals, where the neurons and their relationships remain unknown.
In essence, understanding something as simple as C. Elegans could give us a piece of a neuroscience Rosetta Stone for better understanding other animals, including ourselves.
He who controls the spice might control the universe, but whomever understands the effect of spices on worms, better understands themselves, and that might be better.